Diversity of quinoa genetic resources for sustainable production: A survey on nutritive characteristics as influenced by environmental conditions
- 1Gene Bank, Crop Research Institute, Prague, Czechia
- 2Faculty of Tropical AgriSciences, Czech University of Life Sciences Prague, Prague, Czechia
- 3Quality of Plant Products, Crop Research Institute, Prague, Czechia
Environmental extremes and climatic variability have enhanced the changes in numerous plant stressors. Researchers have been working to improve “major” crops for several decades to make them more adaptable and tolerant to environmental stresses. However, neglected and underutilized crop species that have the potential to ensure food and nutritional security for the ever-growing global population have received little or no research attention. Quinoa is one of these crops. It is a pseudocereal, considered a rich and balanced food resource due to its protein content and protein quality, high mineral content, and health benefits. This review provides currently available information on the genetic resources of quinoa and their quality in terms of variability of economically important traits such as yield, and the content of bioactive compounds, such as protein and amino acid composition. The influence of variety and environmental conditions on selected traits is also discussed. The various types of nutrients present in the different varieties form the basis and are key for future breeding efforts and for efficient, healthy, and sustainable food production.
Introduction
Most staple foods comprise grain crops; therefore, feeding the ever-increasing global population means increasing the production of these crops (Bvenura and Kambizi, 2022). But it is well known that climate change is rapidly degrading the conditions of crop production. Salinization and aridity are forecasted to increase in most parts of the world (Choukr-Allah et al., 2016). Moreover, globally, the food crisis is mainly triggered by shocks such as drought and escalated by trade restrictions leading to price rises as an impact of the Covid-19 pandemic and as a consequence of the current war in Ukraine (Rahut et al., 2022).
As a consequence of this reality, new stress-tolerant or new alternative crops or species must be identified and used for future food security (Choukr-Allah et al., 2016). The present situation is that common wheat, rice, and maize as major crops seem to be near 80% of their potential. This shows the potential of many small-scale and marginal crops and wild plants that can be used as high-quality food sources. Since many of these species are well adapted to extreme environments, their role in the current scenario of climate change has become extremely important (Chrungoo and Chettry, 2021).
These crops have the potential to complement the major cereals and play a greater role in a safe household diet. A better understanding of these crops that feed the world and their potential role in nutrition will help secure their future and ensure food and nutrition security. Chenopodium quinoa Willd. was selected as one of the crops that will contribute to food security in the twentifirst century, because of its high resilience to extreme environmental conditions and its qualities as a functional food (Bvenura and Kambizi, 2022; Singh et al., 2022) and a potentially strategic crop that plays a vital role in food security and sovereignty (Rojas et al., 2015). In addition, quinoa has gained importance in international consumer markets in the last decade, which provides economic opportunities for Andean producers (Anaya et al., 2022). On the other hand, quinoa could be used for crop diversification in Europe and other parts of the world, outside of its genetic origin, as an alternative for marginal agricultural land (Jacobsen, 2017).
In the present work, we attempt to summarize the available information about quinoa genetic resources for the whole world by highlighting the situation in the Czech Republic. We also explore the results of current research focused on nutraceutical properties, including carbohydrates, lipids, proteins, amino acids, vitamins, and minerals. This overview provides an insight into the enormous variability of morpho-phenological traits and nutritive components which are possessed by quinoa germplasm cultivated in different global conditions and shows us how important it is to conserve and protect this richness.
Conservation of global quinoa genetic resources and history of research on quinoa in the Czech Republic
Quinoa plant genetic resources are essential for food and nutrition security and sovereignty of peoples, and they make a significant contribution to meeting the basic needs of humanity. They are part of ancestral and cultural heritage, especially for the countries of the Andean region. Their conservation and sustainable use are therefore the responsibility of society as a whole (Rojas et al., 2015). Quinoa is one of the underutilized crops with public breeding or evaluation programmes in South American countries such as Peru, Ecuador, and Bolivia (Galluzzi and Noriega, 2014). Quinoa seeds of different accessions are currently being conserved in several gene banks around the world (ex situ conservation). However, conservation of agrobiodiversity means conservation of the associated culture, that of indigenous farmers living in the Andean region (Bazile et al., 2016a; Jacobsen, 2017). Thus, although the importance of gene banks for biodiversity conservation is well known, the success of future conservation and breeding programmes depends on the transfer of knowledge and associated practices that can help to adapt quinoa to new regions (Ruiz et al., 2014).
Quinoa germplasm and its wild relatives are estimated at 16,422 accessions worldwide; it is held in 59 institutions (universities, gene banks, research, and agricultural institutions) in 30 countries around the world. 88% of accessions are conserved within the Andean region. The largest collections of quinoa and its wild relatives are held by institutions in Bolivia and Peru, with more than 6,000 accessions (Rojas et al., 2015). Compared to published data from many years ago about quinoa accessions conserved in gene banks (Jacobsen and Mujica, 2002), the collection, characterization, and evaluation of quinoa genetic resources have greatly improved in recent years.
According to available data, the genetic resources of quinoa conserved in collections outside the Andean region comprise a total of 2,137 accessions (Table 1). In the database, the biological status of 1,329 accessions is indicated as traditional cultivar/landrace, 552 accessions are listed as wild, while 1,007 accessions are shown as advanced/improved cultivar, and 100 accessions as others (Genesys, 2022). The provenance of accessions is mostly Peru, followed by the USA and Bolivia. In 1,329 accessions the type of germplasm storage is not identified, 543 genetic resources are kept in as long-term seed collection, 193 are conserved in seed collection, and 45 accessions in the short-term collection. In total, 478 accessions have safety duplication in Svalbard Global Seed Vault in Norway and 143 accessions in National Seed Storage Laboratory, USDA-ARS in the USA. Most of the accessions (1,306) are conserved in the International Center for Biosaline Agriculture in the United Arab Emirates. In Europe, the largest collection (528 accessions) is held by the Genebank of Leibniz Institute of Plant Genetics and Crop Plant Research in Germany (Eurisco, 2022).
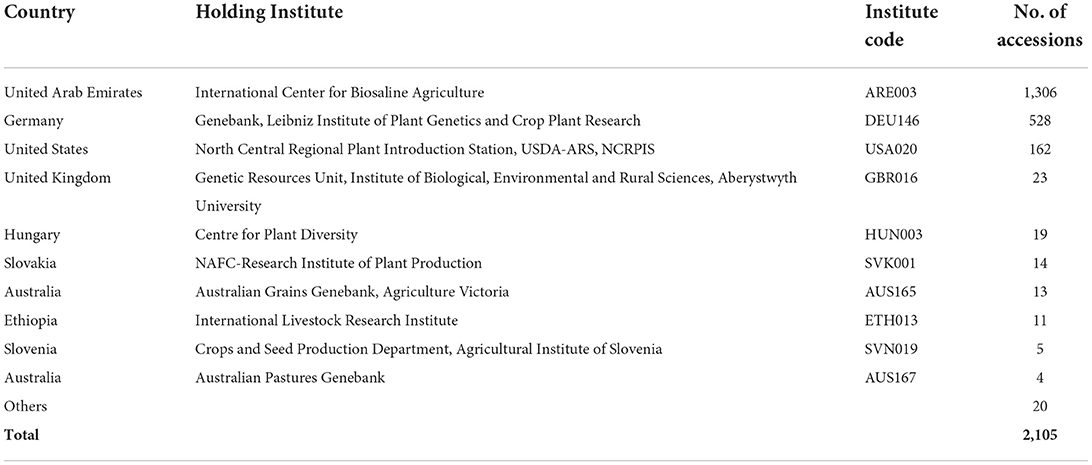
Table 1. Quinoa genetic resources in collections outside the South American region (Genesys, 2022).
In the Czech Republic, research on quinoa genetic resources began in 1999 with Dr. Anna Michalová, who obtained 22 quinoa genotypes from South America. Subsequently, a working collection of quinoa genotypes was established in the gene bank of the Crop Research Institute in Prague. The quinoa accessions were evaluated under field conditions for selected agro-morphological traits (days to flowering, days to harvest, 1,000-seed weight, etc.), and selected nutritional components in the seeds (crude protein content) were also analyzed in the laboratory. Evaluation of the quinoa working collection was stopped until 2016 when Dr. Dagmar Janovská and Dr. Petra Hlásná Cepková resumed work on quinoa genetic resources cultivated under the conditions of the Czech Republic. Currently, the working collection of quinoa includes 70 genotypes. They are being tested under field conditions using descriptors for quinoa and its wild relatives (Bioversity International et al., 2013) while analyses are being conducted in the laboratory to determine the nutritional quality of the seeds of each genotype. The promising material will be used for future breeding purposes.
Global production of quinoa
At present, quinoa is grown throughout North and South America, Europe, Asia, Africa, and Oceania (Hinojosa et al., 2021). Alongside South American countries, China, India, and some European countries cultivate quinoa (Bazile and Baudron, 2015; Mosyakin and Schwartau, 2015; Yang et al., 2019). However, the biggest world producers remain countries of the traditional region of quinoa cultivation: Peru, with the production of 100,115 t; Bolivia, with 70,170 t (Faostat, 2022); and Ecuador, with more than 4,500 t (Hinojosa et al., 2021), while the United States is the top importer (Bvenura and Kambizi, 2022). The global harvested area of quinoa almost doubled last decade from 95,979 ha in 2010 to 188,878 ha in 2020. Annual production in China was 20,000 t in 2018 and harvested area reached nearly 12,000 ha (Yang et al., 2019). Globally, the average yield slightly increased from 0.83 t.ha−1 in 2010 to 0.93 t.ha−1 in 2020 (Faostat, 2022). For example, in Ecuador, the obtained yield in the variety comprises 66% of the total quinoa area which is 1.30 t.ha−1 (Hinojosa et al., 2021).
In the last decade, quinoa has evolved from being a neglected traditional food to an important export crop, promoted as a “superfood” throughout the Western world (Bazile and Baudron, 2015; Nuñez De Arco, 2015). Rising demand among Western consumers has created new economic opportunities for quinoa farmers in Bolivia's southern Altiplano. The negative aspect of the high interest in quinoa and the extreme increase in demand for quinoa seeds is that it has caused a spectacular increase in market price (Tschopp et al., 2018). However, this quinoa boom has brought environmental disaster in the traditional regions of quinoa cultivation in Bolivia (Jacobsen, 2011). Similarly, in Peru, the area under quinoa cultivation has been expanded by 264% and its cultivation has spread to all regions of Peru (Bedoya-Perales et al., 2018) which had a strong negative impact on the environment – soil degradation, pest, and diseases occurrence; likewise on socio-economic links and relations in local communities (Jacobsen, 2011). In the context of the above-mentioned facts, countries of the Andean region have tried to make a great effort to establish a harmonious interaction between socio-economic and environmental demands (Bedoya-Perales et al., 2018) and apply strategies for saving quinoa diversity, established breeding and research priorities, built more transparent commercial chain policy, and ensure more efficient cooperation with local farmers and cooperatives to decrease the negative impact of quinoa growth expansion (Ruiz et al., 2014; Bazile and Baudron, 2015; Bazile et al., 2016a; Bedoya-Perales et al., 2018; Hinojosa et al., 2021).
Quinoa's adaptability to a diverse environment
In different countries around the world, farmers and researchers have been trying to find, test and introduce nutritionally valuable seed crops that would be suitable for diverse growing conditions, achieve satisfactory yields, and offer versatile applications in food production and consumption (Gardner et al., 2019; Toderich et al., 2020; Habiyaremye et al., 2022).
To fully exploit the potential of the crop for marginal environments, identification of new and high-yielding quinoa genotypes with good local adaptation and high nutritional quality are crucial, which requires intensified screening and adaptation research (Choukr-Allah et al., 2016).
Recently, the performance of different quinoa genotypes in different global environments with an emphasis on their adaptability and seed nutritional quality has been studied in many countries and regions (Table 2). The considerable variability in yield for different quinoa genotypes in the different environments was confirmed outside of the Andean region. The lower yields were observed at 0.08 t.ha−1 in Morocco (Taaime et al., 2022) and the highest at 7.86 t.ha−1 (Thiam et al., 2021) also in Morocco. The range of yield in experimental fields of the Czech Republic in 2018–2021 was estimated between 0.12 and 3.99 t.ha−1 (unpublished data). Observed yield levels in Northern Europe were 1–3 t.ha−1 (Pulvento et al., 2012; Jacobsen, 2017; Prager et al., 2018; De Bock et al., 2021b; Granado-Rodriguez et al., 2021b; Matías et al., 2021). However, quinoa yields over the years have remained unpredictable and very low, averaging between 1.2 and 1.4 t.ha−1 while the maximum attainable yield can be up to 8–10 t.ha−1 in Morocco. A range of factors was suggested as affecting production, such as the choice of cultivars, optimal sowing date, and nutrient management was suggested affecting the production (Choukr-Allah et al., 2016). In the same way, high salinity can reduce the yield significantly (Hussain et al., 2020). Grain yield was more influenced by the environment and the genotype-environment interactions. The results of (Thiam et al., 2021) confirmed the significance and challenge of evaluating the varietal grain yield stability across contrasting environments.
The marginal effect of salt stress on nutritional composition was presented by (Choukr-Allah et al., 2016), whereas (Hussain et al., 2020) reported a significant impact of salt stress on grain protein contents dependent on genotype. However, the salinity common in these regions promotes growth but up to a certain threshold, beyond which growth and productivity start to be negatively affected (Choukr-Allah et al., 2016). In testing 20 quinoa genotypes in two different environments in Rwanda, it was confirmed that low water availability affected the growth and yield of quinoa and there is a need to identify the best genotypes adapted to specific agro-ecological zones and even growing seasons (Habiyaremye et al., 2022).
Rising temperatures are challenge for quinoa as well as for other crops. High temperatures during flowering and heat stress during the vegetative stage in certain quinoa varieties considerably lowered yield and changed protein and fiber content (Matías et al., 2021). In the growing conditions of Chile, the influence of increased night temperature on quinoa plants was evaluated (Lesjak and Calderini, 2017). Grain yields were reduced in the range of 12–31% by increased night temperatures. Similarly, the aboveground biomass was affected negatively in contrast with values for harvest index, individual grain weight, grain protein content, and water-soluble carbohydrates, which have changed only slightly.
In Chile, the local landrace genotype Cahuil had the best performance regarding seed yield under water stress (Pinto et al., 2021). Further, the genotype Titicaca (originating from the Andes) showed a good adaptation to the Mediterranean environment with tolerance to salinity and drought (Pulvento et al., 2012). On the other side, in some regions of southeast China, the combination of the high temperatures and heavy rainfalls had negative effects on the growth of quinoa. Fortunately, quinoa germplasm collected from Taiwan showed resistance to high temperatures and heavy rainfalls (Yang et al., 2019). In quinoa growing in the conditions of Morocco, optimal temperatures (10–25°C), high and well-distributed precipitation, and short photoperiods contributed to better growth and the highest yield (Taaime et al., 2022). The susceptibility of quinoa to temperatures above 32°C was confirmed due to the flower closing during the day and limited pollination caused a reduction of the yield by up to 86% (Tovar et al., 2020).
The high degree of variability in the performance of nutritional profiles of quinoa seeds under various salinity stress was assessed while the nutritional value of seeds remained unchanged, especially the high protein content, all essential amino acids, high mineral content, and flavonoids (Pulvento et al., 2012; Toderich et al., 2020). On the other hand, high temperatures increased protein and fiber content (Matías et al., 2021).
However, the establishment of this crop in many agronomical areas outside South America is still limited. It could be considered that the quinoa cultivar selection process remains unfinished for new cultivation areas, including those located in southern Europe which are characterized by having intense precipitations at early growth stages and high temperatures at later stages of crop development (Granado-Rodriguez et al., 2021b). There is still very limited information regarding the stability of seed nutritional characteristics under changing environments (Granado-Rodriguez et al., 2021b).
As with any other new crop, one of the key factors for the successful introduction and establishment of quinoa under new climatic conditions will be the identification of appropriate planting material. Therefore, it is important to study the adaptation and yield of several potential quinoa genotypes from different provenances to select the most promising ones suitable for the local agro-climatic conditions (Choukr-Allah et al., 2016). Not only should adaptation of quinoa be discussed, but also sustainable establishment in a new environment.
Nutritional characteristics of quinoa seeds and plants
Quinoa has outstanding nutritional value in all its edible parts – seeds and leaves, which were recognized even by ancient populations that considered quinoa a sacred food (Jacobsen et al., 2003). Quinoa seeds are a superior source of vitamins, minerals, dietary fiber, and lipids with the presence of health-beneficial polyunsaturated fatty acids (Repo-Carrasco et al., 2003). As reported by Schlick and Bubenheim (1996), quinoa is one of the single food sources that can supply all essential macro and micronutrients required for balanced human nutrition.
Carbohydrates, starch, and total dietary fiber
Quinoa seeds contained a relatively high amount of carbohydrates, with the content ranging from about 42% reported in the variety “Roja” up to 83% found in accessions cultivated in Peru (Encina-Zelada et al., 2017). As summarized in Table 3, there are significant differences in carbohydrate content in various genotypes. For example, Miranda et al. (2012) detected higher carbohydrate content in Chilean highland ecotypes as opposed to southern ecotypes. Pereira et al. (2019) reported slightly higher mean carbohydrate content in black and white varieties but lower in red varieties. In spite of that, many other variables modify total carbohydrate content, such as environmental conditions and sowing date. For example, in sea level genotypes and one cross genotype cultivated in Argentina, winter sowing at 18°C resulted in expanded seed weight, and therefore higher carbohydrate content in seeds (Curti et al., 2018). On the other hand, high carbohydrate content negatively affects total protein content (Craine and Murphy, 2020; De Bock et al., 2021a,b).
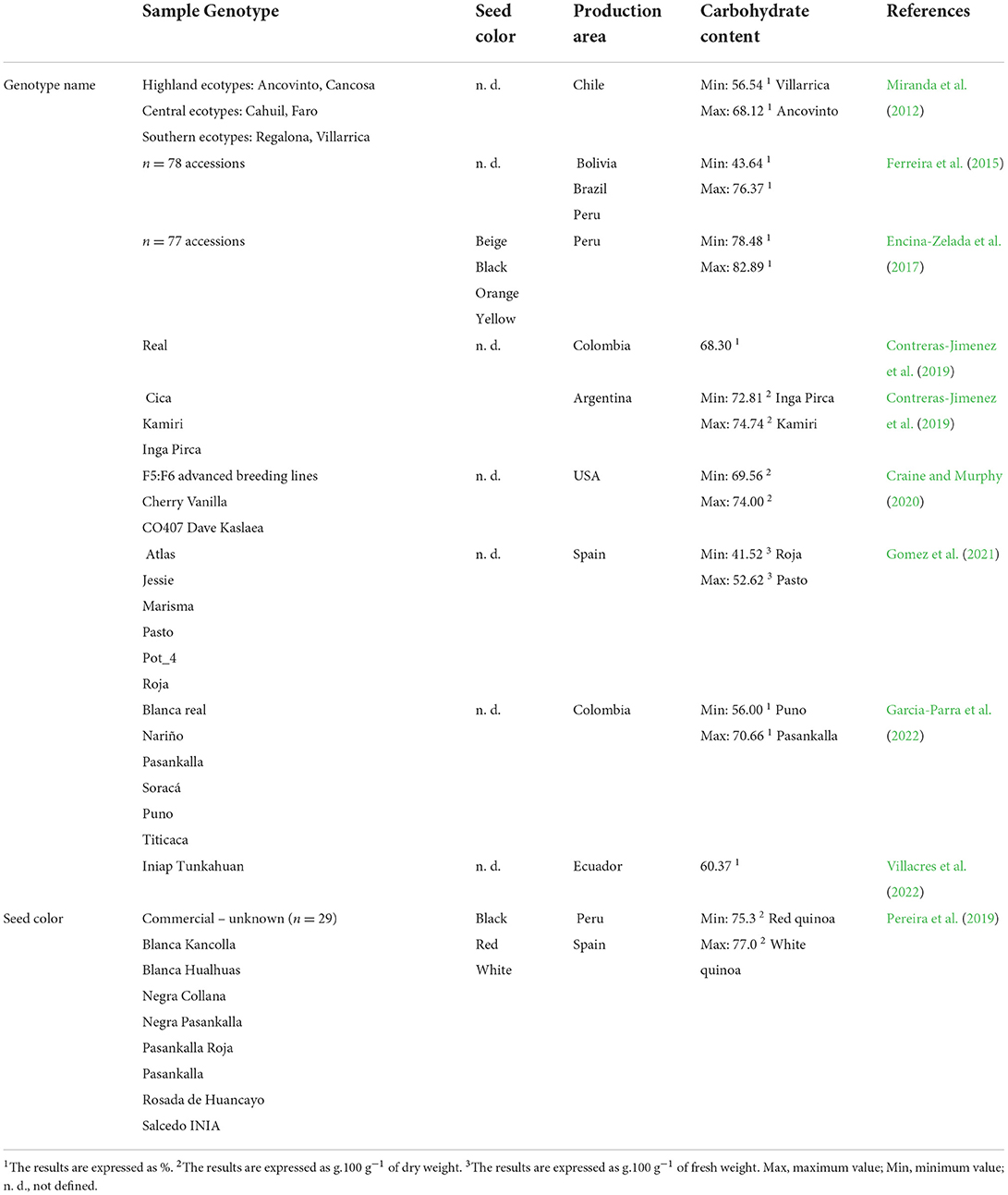
Table 3. Variability of the carbohydrate content in quinoa seeds divided according to the genotype name and seed color.
In terms of environmental influence, increased carbohydrate content was reported for lowland/coastal quinoa genotypes “Regalona Baer” and “Villarrica” in arid conditions with lower soil organic matter content and a mean temperature of approximately 18°C during the growing season (Miranda et al., 2013). Experiments conducted with genotypes cultivated in Spain resulted in decreased carbohydrate content in a growing season with a mean temperature of approximately 25°C, in contrast to a growing season with a mean temperature lowered by 5°C (Matías et al., 2021). This was also supported by Garcia-Parra et al. (2022), indicating the highest carbohydrate content (65.5%) in cultivars grown in a cold climate.
The most prevailing component of quinoa carbohydrates is starch, situated primarily in the perisperm, in contrast to the cereals (Burrieza et al., 2014). The minimal value for starch content was 44%, found in genotype “Cica” (Jimenez et al., 2019) cultivated in Argentina, whereas the most abundant starch content of 72.5% was described by (De Bock et al., 2021b) in genotype “Titicaca” grown under North-West European field conditions. Nonetheless, the values for total carbohydrate content in this study varied between different years of field experiments. Similarly, (Grimberg et al., 2022) characterized the genotype “Titicaca” as one with the most prominent starch content. (Aluwi et al., 2017) evaluated maximal starch content in genotype “CO 407D” [64% in dry weight (dw)] and the lowest for “UDEC-1” (55%), both cultivated in the USA.
Quinoa starch is rich in polysaccharide amylopectin, which represents 54–85% of dw (Dong et al., 2021; Kheto et al., 2022). Amylose content is, on the other hand, relatively low. It ranges from approximately 6% in “Tianjing Tibet Quinoa” (Li and Zhu, 2017) up to 20% in the Argentinian variety “Jujuy” (Nascimento et al., 2014). Specific starch and amylopectin structure give quinoa starch various functional properties that can be used in a wide range of food products (Li et al., 2016; Aluwi et al., 2017; Li and Zhu, 2017). Nevertheless, climatic conditions during the growing season may alter final functionality, even though starch biosynthesis is determined primarily by genetics (Garcia-Parra et al., 2021, 2022). Additionally, seed color seems to correlate with starch physiochemical properties, as reported by Peng et al. (2022), in opposition to Li et al. (2016), describing no correlation between the seed color and starch characteristics.
Total dietary fiber (TDF) content in quinoa is also highly heterogenous, ranging from approximately 7% (De Bock et al., 2021a) up to 23% (Granado-Rodriguez et al., 2021b). The variation can be explained by the genotype effect (Curti et al., 2018), but also by growing conditions since fiber content can be enhanced under saline conditions (Pulvento et al., 2012) and high temperatures during the grain filling period (Matías et al., 2021). Negative correlations were found between TDF, carbohydrate, and fat content (Vidueiros et al., 2015). Overall, high amounts of TDF (over 18% TDF) were found in genotypes “Rainbow”, “Faro”, “Baer”, “Colorado 407D” cultivated in Poland (Sobota et al., 2020), “Titicaca” grown in Italy (Pulvento et al., 2012), and “Roja” and “Duquesa” grown in Spain (Matías et al., 2021). Less prominent amounts (below 14% TDF) were presented in “Faro Red”, “Puno” (Sobota et al., 2020), “Pasto” (Matías et al., 2021), white Bolivian and Peruvian quinoas (Pellegrini et al., 2018), “Cica”, “Kamiri” and “Inga Pirca” (Jimenez et al., 2019).
Protein content and amino acid composition
Quinoa seeds are often considered high in protein; yet overall protein content is quite variable (Table 4) and sometimes comparable to or higher than in most cereals such as wheat (12%), oat (13%), rice (7%), and corn (6%) (USDA, 2020). Variations in protein content were significant in several genotypes cultivated in distinctive agro-ecological conditions. For example, the cultivar “Jessie” originating in France was cultivated in Belgium and reached almost 19% protein content (De Bock et al., 2021b), whereas the same genotype cultivated in Germany reached a protein content of approximately 12% (Prager et al., 2018). Nevertheless, “Jessie” cultivated for two years in southwest Spain showed a steady mean protein content of 16.7% (Matías et al., 2021).
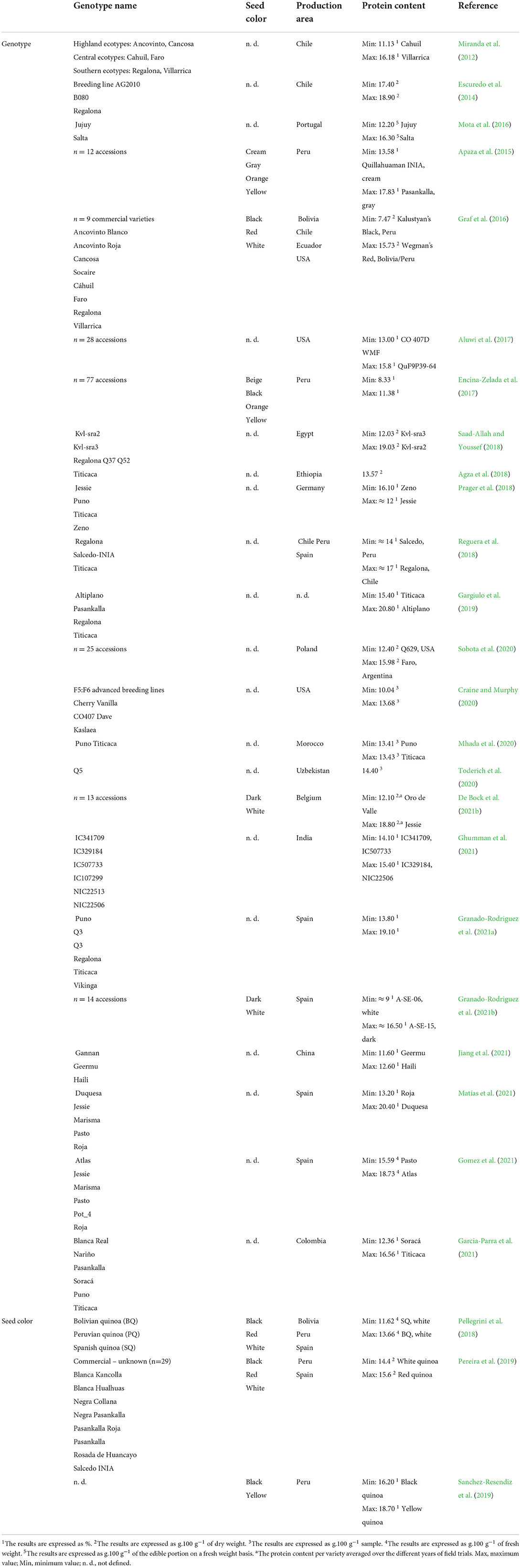
Table 4. Variability of protein content in quinoa seeds divided according to the genotype and seed color.
The Danish-bred cultivar “Titicaca” was analyzed in at least 10 studies under distinctive environmental conditions. Despite that, this genotype reached analogous values (13–15%) in the cultivation conditions of Ethiopia (Agza et al., 2018), Morocco (Mhada et al., 2020), Belgium (De Bock et al., 2021b), USA (Aluwi et al., 2017), and Germany (Prager et al., 2018). Besides this, slightly higher protein content (above 15%) was observed under cultivation in Poland (Sobota et al., 2020) and Colombia (Garcia-Parra et al., 2022). In addition, Reguera et al. (2018) reported higher protein content for “Titicaca” cultivated in Chile compared to Spain, which follows the results of Granado-Rodriguez et al. (2021a), reaching comparable values in mean protein content averaged for three cultivation years.
Genotype “Regalona”, originating in southern regions of Chile, was described in at least eight studies. The values for protein content were quite inconsistent. Miranda et al. (2012), Graf et al. (2016), and Granado-Rodriguez et al. (2021a) detected protein content reaching approximately 13–15% for “Regalona” cultivated in Chile and Spain, whereas other authors achieved higher values of approximately 17% under field experiments in Chile and Egypt (Lesjak and Calderini, 2017; Reguera et al., 2018; Saad-Allah and Youssef, 2018). Even higher values were achieved by Gargiulo et al. (2019) (18.30%); however, the authors did not define the cultivation location.
The protein content of the Danish cultivar “Puno” was described in at least seven studies. The majority of the results were quite consistent in diverse environments (USA, Germany, Poland, Belgium, Colombia), ranging between 13 and 15% (Aluwi et al., 2017; Sobota et al., 2020; De Bock et al., 2021b; Garcia-Parra et al., 2022). On the other hand, (Garcia-Parra et al., 2021) evaluated slightly reduced protein content, reaching almost 12% in “Puno” cultivated in Colombia.
Although the Peruvian genotype “Pasankalla” was tested in at least 4 studies, the referred values of protein content are quite distant. Apaza et al. (2015) and Gargiulo et al. (2019) discovered protein content of 18.73–20.60%, while Garcia-Parra et al. (2021) and Garcia-Parra et al. (2022) achieved lower values (14.5–15.5%, respectively) during experiments conducted in Colombia. Genotype “Cahuil” originating in central Chile was investigated in a total of three studies. Miranda et al. (2012) reported protein content of 11.13%, whereas Graf et al. (2016) presented a lower concentration of nearly 9%. Aluwi et al. (2017) recognized a much higher protein content of 14.4% under cultivation in the USA.
Nonetheless, there are many factors affecting the resulting protein content. Besides the influence of genotype, the importance of soil matric potential (SMP) and nitrogen fertilization was indicated (Wang et al., 2020). High SMP values (over −55 kPa) cause significant water stress and may also limit nitrogen uptake, which concurs with other studies (Sun et al., 2014; Walters et al., 2016). Therefore, to reach optimal protein content, irrigation is crucial for some genotypes cultivated in adverse soil-water conditions, although slight water stress may enhance protein content (Wang et al., 2020). The intense application of nitrogen from 80 to 240 kg/ha increased protein content by approximately 1.5%. The positive effect of nitrogen fertilization was also presented by Wu et al. (2016) and Jacobsen and Christiansen (2016).
In addition, protein content in quinoa rises under salinity treatment, which was reported for varieties “CO407D”, “UDEC-1”, “Baer”, “QQ065” (Wu et al., 2016), and “NSL106398” (Hussain et al., 2020). In contrast, Ruiz et al. (2016) expressed a drop in protein content by 7–12% in coastal lowland Chilean landraces (“VI-1”, “Villarrica”) and genotype “R49” (salares ecotype). In terms of temperature influence, protein content under heat stress was outstanding in varieties “Pasto”, “Marisma”, “Jessie”, “Roja”, and “Duquesa” (Matías et al., 2021). Garcia-Parra et al. (2022) detected slightly higher mean protein values for cultivation in the cold climate of Colombia, compared to temperate and warm conditions; but, as reported by the authors, protein content was not rapidly affected by elevated temperatures. The exception in this paper was the cultivar “Pasankalla”, showing a decline in protein content in hotter conditions.
Those results suggest the great potential of the selected quinoa genotypes for cultivation in adverse environments. A high correlation was detected between embryo weight ratio and protein content since proteins are mostly stored in the embryo (Gargiulo et al., 2019). Protein content negatively correlates with panicle height and panicle biomass, whereas positive correlations were determined for total phenolic content, antioxidant activity, and saponin content (Granado-Rodriguez et al., 2021b).
Probably even more important than overall protein content is the quality of protein, given by the composition of essential amino acids (EAA). Quinoa protein generally contains all EAAs and several authors throughout the literature have concluded that quinoa protein is complete due to the superior composition of amino acids (AA; Nowak et al., 2016; Maradini et al., 2017; Schmidt et al., 2021). Nonetheless, Craine and Murphy (2020) argue that many of those studies evaluated outdated daily requirements or considered AA requirement values only for adults, not for children, whose requirements for EAAs are greater. The authors further stated that the quinoa protein is only “nearly complete”. Regarding this statement, Boye et al. (2012) labeled valine and lysine as limiting AA for children up to the age of 10 years. In comparison, Gonzalez et al. (2012) suggested lysine, tyrosine, and tryptophan as limiting AA for the age group of 2–5 years. Craine and Murphy (2020) identified low leucine content, which does not achieve the recommended daily requirements for infants and children, therefore considering it as limiting AA.
As expressed in Table 5, the content of each EAA shifted between authors. The most abundant EAA was leucine with the highest content in the variety “Atlas” (Gomez et al., 2021), whereas the least represented EAA was tryptophan, with the content reaching 0.58–1.9 in g.100 g−1 protein in genotypes “Chucapaca” and “Bastille”, respectively (Escuredo et al., 2014; De Bock et al., 2021b). With regards to the previously mentioned limiting AAs, several genotypes accomplished the daily requirements for EAAs in infants and children (WHO/FAO/UNU, 2007). As such, sufficient lysine content (over 5.7 g.100 g−1 protein) was identified in genotypes “Jessie”, “Pasto”, and “CICA”. Valine content (over 4.3 g.100 g−1 protein) was satisfactory in genotypes “Ancovito”, “CICA”, “Jessie”, “Rouge Marie”, “Zwarte”, and “Roja”. Suitable leucine content (over 6.6 g.100 g−1 protein) was found in genotypes “Villarrica”, “Rataqui”, “Atlas”, and “Jessie”. Tryptophan content (over 0.85 g.100 g−1 protein) was met in genotypes “Sajama”, “B080”, “Regalona”, “Zeno”, “Puno”, and all genotypes analyzed by De Bock et al. (2021b) (Table 5).
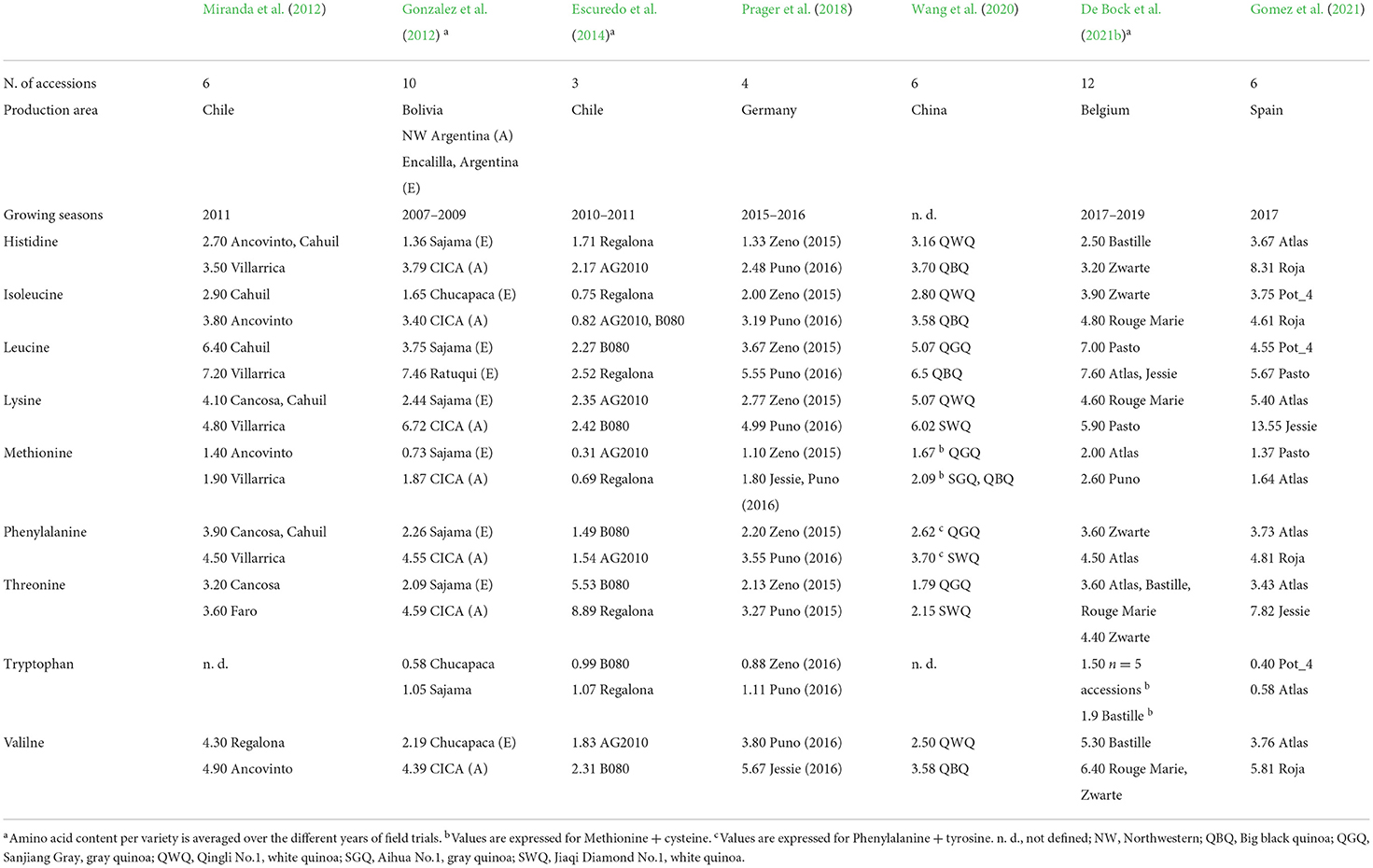
Table 5. Minimum and maximum values of amino acid composition (g.100 g −1 protein) in various quinoa genotypes and production areas.
Overall, the remarkable variations in EAA composition might be caused by genotype, environment, and their interactions. According to De Bock et al. (2021b), the content of EAAs varied between growing seasons, but not between varieties, in contrast to Prager et al. (2018), who noticed significant differences among cultivars and experimental years. In terms of cultivation area, Steffolani et al. (2016) pointed out that Bolivian varieties had higher essential AA content than Peruvian varieties. Gonzalez et al. (2012) indicated dissimilarities in AA content between two experimental sites with higher EAA content in the Bolivia/Argentina location, which authors then explain by adaptation of the genotypes to the conditions they were bred in. Reguera et al. (2018) noted that varieties grown in Chile did not exhibit inter-cultivar variations in AA content compared to the same varieties grown in Spain, except for cultivar “Titicaca” which had consistent AA content among varieties and locations. The highest EAA content in genotypes cultivated in the USA was recognized in samples from the Chimacum location, as opposed to Mount Vermont samples (Craine and Murphy, 2020).
Most of the EAAs were not negatively affected by salinity in “Q5”, a new salt- and drought-tolerant line, except for tyrosine (Toderich et al., 2020). Aloisi et al. (2016) found variations in genotype response to saline conditions. EAAs remained constant or declined, except for increased methionine in genotype “R49”, belonging to the group of salares ecotype; and leucine in genotype “Villarrica” (coastal-lowland ecotype). A strong decline in EAAs under salinity treatment was detected in genotype VI-1 (coastal-lowland ecotypes). Despite this, Ruiz et al. (2016) concluded better suitability of “VI-1” and “Villarrica” in saline environments in terms of growth, yield, phenolic content, and protein profiles compared to the “R49”; however, other nutritional characteristics were not studied in this paper. Therefore, the selection of saline-resistant genotypes and the analysis of nutritional modifications under stress are crucial.
An essential factor in protein quality evaluation is digestibility. The information about protein digestibility in available scientific literature is sparse and often outdated. For example, Ruales and Nair (1992) reported the true protein digestibility of raw and washed quinoa reaching almost 92%. In addition, the biological value of quinoa protein (above 80%) was considerably higher compared to common cereals or soybean. On the other hand, significantly lower protein biological values were reported by Paucar-Menacho et al. (2018). Recently, Shi et al. (2020) reported the in vitro protein digestibility (IVPD) in quinoa ranging from ~73 to 79% with in vitro protein digestibility corrected amino acid scores (IV-PDCAAS) of 48–57%. Authors reported lower values in cultivar “NQ94PT”, compared to the commercial blend of cultivars “Kankolla” and “Blanca Juli”. Further, Jimenez et al. (2019) reported quinoa IVPD of ~61–63% in varieties “Cica”, “Kamiri”, and “Inga Pirca” obtained from Argentina. In addition, Craine and Murphy (2020) evaluated the PDCAAS in varieties “Colorado D407” ranging from 0.74 to 0.90 and 0.78 to 0.95 for the 1–2 an10-year-old children, respectively.
Overall protein digestibility can be improved by various processing methods (Rizzello et al., 2016; Lorusso et al., 2017; Dong et al., 2021; He et al., 2022), as well as sprouting (Jimenez et al., 2019). On the other hand, digestibility is reduced by the presence of starch, fiber (Opazo-Navarrete et al., 2019), and various antinutritional compounds (Gilani et al., 2012).
Lipid content and composition
Lipid content is, among other factors, strongly affected by genotype (Curti et al., 2020; Garcia-Parra et al., 2022). Since the primary lipid storage is located in the embryo, embryo size may also correlate to overall seed lipid content (De Bock et al., 2021b). The highest lipid yield was described in the genotype “Yellow Marangí”, cultivated in Peru, reaching almost 10% (Apaza et al., 2015), whereas the lowest lipid content reached nearly 3% in quinoa variety “QU5”, cultivated in Belgium (De Bock et al., 2021a) and commercial variety “Gramolino” from Ecuador (Graf et al., 2016; Table 6). In addition, colored seed samples tend to exhibit higher lipid content than white seed samples (Pellegrini et al., 2018); yet Tang et al. (2015) and Shen et al. (2022) obtained the opposite findings. Overall oil content was negatively correlated to protein content (Matías et al., 2021).
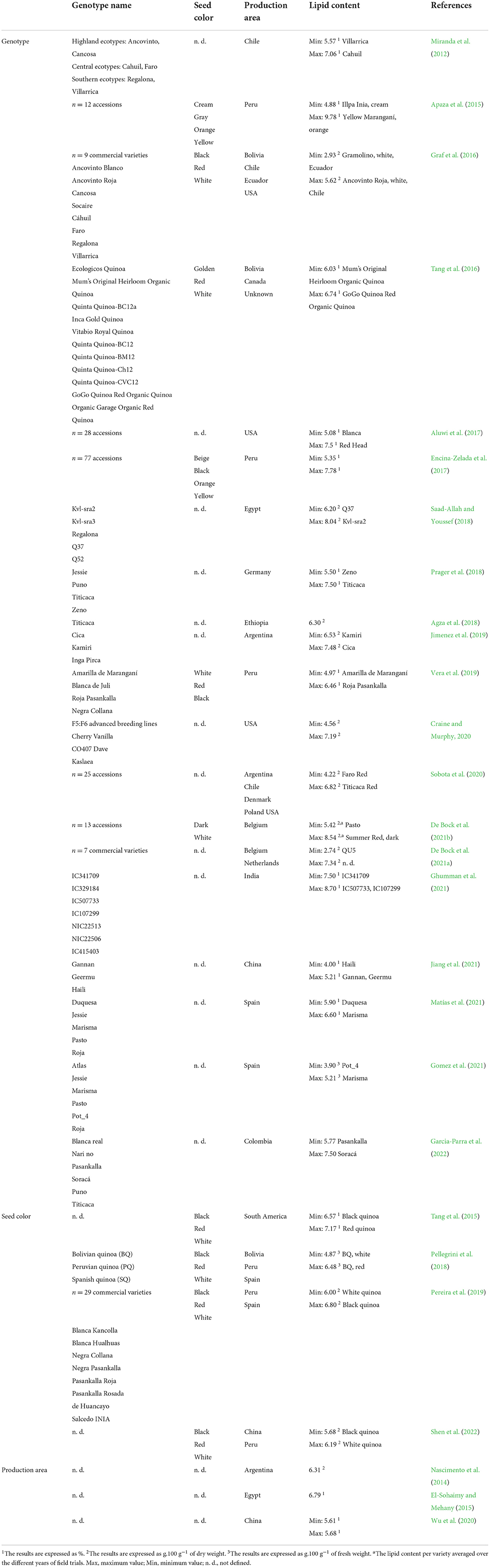
Table 6. Variability of lipid content in quinoa seeds divided according to genotype name and seed color.
In terms of oil production, quinoa performed well in a temperate climate since heat stress reduced average oil content by almost 30% (Garcia-Parra et al., 2022). Curti et al. (2018) found strong interactions between cultivar and sowing date, related to the various photo-thermal conditions during sowing. In a two-year experiment with cultivars “Titicaca” and “Jessie”, stable results were achieved with a mean crude fat content of 7.5 and 7.3%, respectively (Prager et al., 2018). Unfortunately, there are only a small number of studies on quinoa oil production with regard to meteorological conditions during the growing season and the adaptive response of the genotype.
Quinoa lipid profile is composed predominantly of essential polyunsaturated ω-6 linoleic acid (C18:2), with a minimum of 43% in accession “CHEN 414” originating in dry valleys of North Argentina (Vidueiros et al., 2015) and a maximum value of 63% in variety “Temuko” cultivated in the USA (Chen et al., 2019). Quinoa oil also contains a relatively high volume of monounsaturated oleic acid (C18:1), reaching minimum values of 16% in commercial variety “Quinta Quinoa-BC12” (Tang et al., 2016) and maximum values of 33% in accession “CHEN 465” originating in the transition zone of Northwest Argentina (Vidueiros et al., 2015). Saturated palmitic acid (C16:0) was presented in 3.4–13% in genotype “QuF9P39-73” (Chen et al., 2019) and white quinoa genotype (Tang et al., 2016; Shen et al., 2022), respectively. A negative correlation was found between palmitic acid (C16:0) and oleic acid (C18:1), as reported by (Chen et al., 2019).
Less abundant fatty acid in quinoa lipid profile is an essential ω-3 α-linolenic acid (C18:3), which reaches 4–8% (Tang et al., 2016; De Bock et al., 2021a,b; Shen et al., 2022); yet (Vera et al., 2019) found values reaching 11% in yellow quinoa cultivar. Vidueiros et al. (2015) determined the range for α-linolenic acid as 3.2–9.4% for accessions “CHEN 465” and “CHEN 60”, respectively. Quinoa oil also has several minor fatty acids, such as myristic acid (C14:0), stearic acid (C18:0), behenic acid (C22:0), gadoleic acid (C20:1), arachidonic acid (C20:4), and erucic acid (C22:1); however, those are presented only in negligible amounts (below 2%; Tang et al., 2015; De Bock et al., 2021b; Shen et al., 2022).
Several authors noticed variations in fatty acid profiles between varieties (Tang et al., 2016; De Bock et al., 2021b; Shen et al., 2022), but Prager et al. (2018) did not report any significant alterations between varieties or years. Toderich et al. (2020) indicated changes in fatty acid composition in genotype “Q5” grown in saline soils. While the majority of fatty acids declined in medium salinity, the content of palmitoleic acid (C16:1) and arachidic acid (C20:0) was slightly raised. Besides that, the high mixed salinity of sodium chloride and sodium sulfate resulted in a significant increment of stearic acid (C:18:0). The authors also concluded that sulfate salinity affects the fatty acid composition more than sodium chloride type of salinity.
Elevated temperature, together with cultivar-specific response, resulted in lower content of some fatty acids, especially oleic acid (C18:1), stearic acid (C18:0), gadoleic acid (C20:1), and behenic acid (C22:0) (Matías et al., 2021). In contrast, the content of linoleic acid (C18:2) increased or remained unaffected in hot conditions in some cultivars (Curti et al., 2020; Matías et al., 2021). In terms of major fatty acid content, genotype “Jessie” with the shortest life cycle performed better in hot conditions compared to other genotypes. A very important role in quinoa oil quality is also played by optimal fertilization since correlations between some minerals and fatty acid content were observed by Matías et al. (2021).
Based on the available scientific literature, black genotypes tend to have higher polyunsaturated fatty acid (PUFA) content as opposed to red or white seed genotypes (Tang et al., 2015; Pellegrini et al., 2018; Pereira et al., 2019; Shen et al., 2022). Moreover, the highest monounsaturated fatty acid (MUFA) and saturated fatty acid (SFA) content were present in red genotypes (Tang et al., 2015; Pellegrini et al., 2018; Pereira et al., 2019; Vera et al., 2019), in contrast to Shen et al. (2022) who obtained opposed outcomes (Table 7). Nonetheless, as discussed in previous paragraphs, the content of fatty acids is strongly affected by genotype x environment interactions.
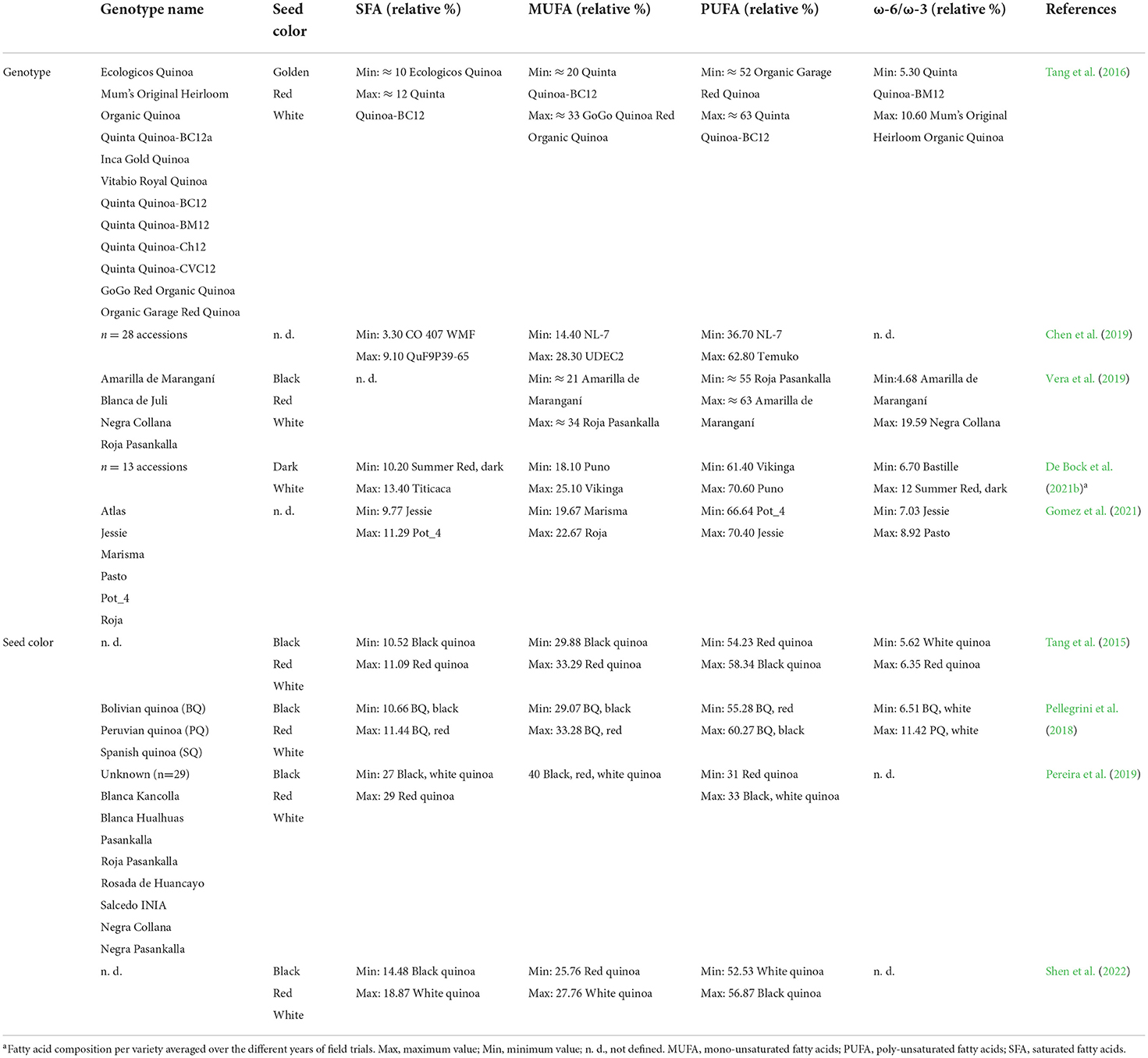
Table 7. Variability of lipid composition in quinoa seeds divided according to the genotype and seed color.
The overall nutritional quality of oils is characterized by the ω-6/ω-3 ratio, with an ideal composition of 1–4/1 in the human diet, as recommended by Simopoulos (2002). Nevertheless, the ω-6/ω-3 ratio of quinoa did not meet the required values since it ranged from 4.7% in variety “Amarilla de Maranganí” up to nearly 20% in variety “Negra Collana” produced in Peru (Vera et al., 2019; Table 7). Despite that, the fatty acid proportion and related nutritional quality are better than in amaranth with values reaching 33–69% (Tang et al., 2016; Paucar-Menacho et al., 2018).
Vitamin and minerals
Quinoa seeds generally contain a sufficient amount of minerals, such as Ca, Fe, Mg, Na, P, K, and Zn (Granado-Rodriguez et al., 2021a,b). As indicated by several authors, quinoa seeds have an even higher content of many minerals than common cereals (Martin et al., 2014; Nascimento et al., 2014; Mhada et al., 2020; Hussain et al., 2021). The content of minerals fluctuates due to genotype, soil type, year, and fertilization (Miranda et al., 2013; Prado et al., 2014; Pellegrini et al., 2018; Granado-Rodriguez et al., 2021a; Bock et al., 2022).
According to Granado-Rodriguez et al. (2021b), the content of P, Ca, and Fe remained unmodified between varieties, as opposed to K, Mg, and Na. Almost equivalent conclusions were defined by Matías et al. (2021), reporting significant fluctuations between cultivars in K and Mg contents, but also in P content, which conflicts with the previous study. Furthermore, Granado-Rodriguez et al. (2021a) stated that the content of Mg, Fe, and Zn was not strongly modified by cultivar x year interactions. Reguera et al. (2018) noticed changes only in Zn between diverse locations, but not within cultivars, whereas De Bock et al. (2021b) recorded no variations in P and Ca content over the years but among the varieties. In addition, no difference between varieties was observed in P, Mg, and Fe concentrations; however, a higher accumulation of P was specific in dark-colored varieties. Higher content of P positively influenced the content of linoleic acid (C18:2) and negatively affected several MUFAs (Matías et al., 2021), which may explain, to some extent, why black seeded varieties contain higher PUFA content than red or white genotypes, as seen in Table 5. Strong correlations were also determined in P and protein content (Granado-Rodriguez et al., 2021b; Matias et al., 2022).
Significant contrasts in mineral concentration between cultivars were also analyzed between hot and cool years, which were probably caused due to little-understood heat-induced adaptation mechanisms and/or interactions among nutrients (Matías et al., 2021). Similar results were also confirmed by Tovar et al. (2020), who highlighted the relationship between heat exposure and specific stages of panicle development. Reguera et al. (2018) investigated aberrations in mineral content between varieties and the agro-ecological conditions they were grown in. According to their findings, the largest accumulation of Mg and Fe in seeds was characteristic of genotypes cultivated in Chile (Río Hurtado). Also, “Regalona” stored a larger amount of almost all analyzed minerals when cultivated in Chile, whereas “Salcedo-INIA” had a larger amount of Mg, Fe, Ca, and Zn when cultivated in Peru (Arequipa). In contrast to that, “Regalona”, cultivated in Chile was characterized as the genotype with the lowest mineral content (Martin et al., 2014).
Genotypes “Pasto”, “Dutchess”, “Atlas”, and “Summer Red” cultivated in Belgium had the highest amount of minerals, in contrast to the other studied genotypes in the experiment of De Bock et al. (2021b). Granado-Rodriguez et al. (2021b) also identified “Pasto”, together with “Marisma”, as genotypes with significantly higher mineral content. On the other hand, Matías et al. (2021) determined “Jessie” as the genotype with the highest mineral content. All genotypes in both studies were cultivated in Spain. In terms of adaptability to adverse conditions, Toderich et al. (2020) referred to the genotype “Q5” as suitable for saline environments since there was a remarkable increment of Fe, Zn, and Ca content under salinity. Mineral concentration varied under contrasting irrigation treatments, except for Mn concentration, which was not significantly different (Walters et al., 2016). The authors also estimated that heterogeneity in concentrations might occur due to the dilution effect.
Although there is not enough current data on overall vitamin content in quinoa, it was concluded in previous studies that quinoa has a satisfactory concentration of thiamine (B1), riboflavin (B2), niacin (B3), pyridoxine (B6), folic acid, and vitamins A, C, and E (Koziol, 1992; Ruales and Nair, 1992). Vitamin E is a general term for tocopherols (α-, β-, γ-, and δ-) and tocotrienols (α-, β-, γ-, and δ-), also named vitamin E homologs. According to Fischer et al. (2013), vitamin E content in quinoa seeds was ranging between 1.04–1.28 mg.100g−1, and overall content was not altered by escalated moisture deficit in genotypes “Regalona”, “B080”, and “AG2010”. Tang et al. (2016) found significant variations in overall vitamin E content and the composition of vitamin E homologs. The most abundant vitamin E homolog in quinoa was γ-tocopherol followed by α-tocopherol, and δ-tocopherol, which is in accordance with the results of Pereira et al. (2019) and Granda et al. (2018). No tocotrienols were detected in any of mentioned studies. Pereira et al. (2019) also determined higher content of γ- and β-tocopherols in the black genotype, but higher α-tocopherol content in the red genotype.
Miranda et al. (2013) uncovered significant alterations in vitamin B content caused by distinct environmental conditions in two studied localities with the highest concentration of B vitamins in the arid locality Vicuña in Chile. Granda et al. (2018) also observed diverse content of vitamin B. While the content of B2 and B6 was relatively similar among varieties, diverse values were determined for B1. The highest concentration of B1 was found in non-pigmented varieties “Tunkahuan” and “Titicaca”. Increased content of B2 appeared in colored varieties and the highest content of B6 was identified in pigmented variety “Pasankalla”. The vitamin C content also shows some changes between distinctive locations with the highest content (49.30 mg 100.g−1 dw) in genotype “Villarrica” cultivated in location Temuco with a cold temperate climate (Miranda et al., 2013).
Summary
This overview provides a summary focused on current research of different quinoa genetic resources in diverse growing conditions. Quinoa is considered a highly nutritive crop that is also resistant to drought and salt suitable for marginal regions. According to our findings, the different environmental condition can have a strong impact on the nutritive compounds of quinoa seeds. Further, the adaptation of quinoa to adverse conditions has limitations in the case of elevated temperatures, high salinity levels, or a combination of weather extremes – heavy rainfall followed by temperatures over 30°C – together with cultivar-response may negatively affect growth and productivity which can result in changed content of nutritive compounds. However, an insight into the enormous variability of nutritive components possessed by quinoa germplasm cultivated in the different conditions of the world shows us how important it is to conserve and protect this richness, and to select outstanding accessions suitable to different conditions. It gives us the potential and hope to develop new varieties of quinoa adapted to different environments and production systems.
Author contributions
DJ and PC: conceptualization. PC and LD: resources and writing–original draft preparation. DJ, MJ, PC, and IV: writing–review and editing. DJ: supervision. DJ, PC, IV: funding acquisition. All authors contributed to the article and approved the submitted version.
Funding
This work was financially supported by the Ministry of Agriculture of the Czech Republic (No. RO0418) and by the Internal Grant Agency of the Faculty of Tropical AgriSciences, Czech University of Life Sciences Prague IGA (Project No. 20223105).
Acknowledgments
We gratefully acknowledge the Subsidy Programme-the National Programme for the Conservation and Use of Plant Genetic Resources and Agrobiodiversity (No. 6.2.5/51834/2017-MZE-17253) for information about quinoa genetic resources in the Czech Republic.
Conflict of interest
The authors declare that the research was conducted in the absence of any commercial or financial relationships that could be construed as a potential conflict of interest.
Publisher's note
All claims expressed in this article are solely those of the authors and do not necessarily represent those of their affiliated organizations, or those of the publisher, the editors and the reviewers. Any product that may be evaluated in this article, or claim that may be made by its manufacturer, is not guaranteed or endorsed by the publisher.
References
Agza, B., Bekele, R., and Shiferaw, L. (2018). Quinoa (Chenopodium quinoa, wild): as a potential ingredient of injera in Ethiopia. J Cereal Sci. 82, 170–174. doi: 10.1016/j.jcs.2018.06.009
Ahmadi, S. H., Solgi, S., and Sepaskhah, A. R. (2019). Quinoa: A super or pseudo-super crop? Evidences from evapotranspiration, root growth, crop coefficients, and water productivity in a hot and semi-arid area under three planting densities. Agri. Water Manage. 225, 105784. doi: 10.1016/j.agwat.2019.105784
Aloisi, I., Parrotta, L., Ruiz, K. B., Landi, C., Bini, L., Cai, G., et al. (2016). New insight into quinoa seed quality under salinity: changes in proteomic and amino acid profiles, phenolic content, and antioxidant activity of protein extracts. Front. Plant Sci. 7, 656. doi: 10.3389/fpls.2016.00656
Aluwi, N. A., Murphy, K. M., and Ganjyal, G. M. (2017). Physicochemical characterization of different varieties of quinoa. Cereal Chem. 94, 847–856. doi: 10.1094/CCHEM-10-16-0251-R
Anaya, R. B., La Cruz, D. e., Munoz-Centeno, E., Condor, L. M., Leon, R. R., and Carhuaz, R. (2022). Food and medicinal uses of ancestral andean grains in the districts of quinua and acos vinchos (ayacucho-peru). Agronomy ,12, 1014. doi: 10.3390/agronomy12051014
Apaza, V., Cáceres, G., Estrada, R., and Pinedo, R. (2015). Catalogue of Commercial Varieties of Quinoa in Peru. A Future Planted Thousands of Years a Ago. Lima: FAAAOOTU Nations. (Lima).
Asher, A., Galili, S., Whitney, T., and Rubinovich, L. (2020). The potential of quinoa (Chenopodium quinoa) cultivation in Israel as a dual-purpose crop for grain production and livestock feed. Sci. Horti. 272, 109534. doi: 10.1016/j.scienta.2020.109534
Bazile, D., and Baudron, F. (2015). “The dynamics of the global expansion of quinoa growing in view of its high biodiversity,” in State of the Art Report on Quinoa Around the World in 2013, eds. D. Bazile, D. Bertero and C. Nieto (Rome: FAO/CIRADE), 44–55.
Bazile, D., Jacobsen, S. E., and Verniau, A. (2016a). The global expansion of quinoa: trends and limits. Front Plant Sci. 7, 622. doi: 10.3389/fpls.2016.00622
Bazile, D., Pulvento, C., Verniau, A., Al-Nusairi, M. S., Ba, D., Breidy, J., et al. (2016b). Worldwide evaluations of quinoa: preliminary results from post international year of quinoa fao projects in nine countries. Front. Plant Sci 7, 850. doi: 10.3389/fpls.2016.00850
Bedoya-Perales, N. S., Pumi, G., Talamini, E., and Padula, A. D. (2018). The quinoa boom in Peru: will land competition threaten sustainability in one of the cradles of agriculture? Land Use Policy 79, 475–480. doi: 10.1016/j.landusepol.2018.08.039
Benlhabib, O., Boujartani, N., Maughan, P. J., Jacobsen, S. E., and Jellen, E. N. (2016). Elevated Genetic Diversity in an F-2, 6. population of quinoa (Chenopodium quinoa) developed through an inter-ecotype cross. Front. Plant Sci. 7, 1222. doi: 10.3389/fpls.2016.01222
Bioversity International FAO, Proinpa, I., and Ifad, A. (2013). “Descriptors for quinoa (Chenopodium quinoa willd.) and wild relatives, in Bioversity International, FAO, PROINPA, INIAF and IFAD. 2013 (Rome: Bioversity International and FAO).
Bock, D. E., Cnops, P., Muylle, G., Quataert, H., Eeckhout, P. M., and Van Bockstaele, F. (2022). Physicochemical characterization of thirteen quinoa (Chenopodium quinoa willd.) varieties grown in north-west Europe-part II. Plants 11:265. doi: 10.3390/plants11030265
Boye, J., Wijesinha-Bettoni, R., and Burlingame, B. (2012). Protein quality evaluation twenty years after the introduction of the protein digestibility corrected amino acid score method. Br. J. Nutr. 108, S183–S211. doi: 10.1017/S0007114512002309
Burrieza, H. P., Lopez-Fernandez, M. P., and Maldonado, S. (2014). Analogous reserve distribution and tissue characteristics in quinoa and grass seeds suggest convergent evolution. Front. Plant Sci 5, 546. doi: 10.3389/fpls.2014.00546
Bvenura, C., and Kambizi, L. (2022). “Future grain crops,” in Future Foods Global Trends, Opportunities, and Sustainability Challenges, ed. R. Bhat (London: Academic Press), 81–105. doi: 10.1016/B978-0-323-91001-9.00032-3
Chen, Y. S., Aluwi, N. A., Saunders, S. R., Ganjyal, G. M., and Medina-Meza, I. G. (2019). Metabolic fingerprinting unveils quinoa oil as a source of bioactive phytochemicals. Food Chem. 286, 592–599. doi: 10.1016/j.foodchem.2019.02.016
Choukr-Allah, R., Rao, N. K., Hirich, A., Shahid, M., Alshankiti, A., Toderich, K., et al. (2016). Quinoa for marginal environments: toward future food and nutritional security in mena and central asia regions. Front. Plant Sci. 7, 346. doi: 10.3389/fpls.2016.00346
Chrungoo, N. K., and Chettry, U. (2021). Buckwheat: a critical approach towards assessment of its potential as a super crop. Indian J. Genet. Plant Breed. 81, 1–23. doi: 10.31742/IJGPB.81.1.1
Contreras-Jimenez, B., Torres-Vargas, O. L., and Rodriguez-Garcia, M. E. (2019). Physicochemical characterization of quinoa (Chenopodium quinoa) flour and isolated starch. Food Chem. 298, 124982. doi: 10.1016/j.foodchem.2019.124982
Craine, E. B., and Murphy, K. M. (2020). (2020). Seed composition and amino acid profiles for quinoa grown in Washington State. Front. Nutr. 7, 126. doi: 10.3389/fnut.2020.00126
Curti, R. N., Sanahuja, M. D., Vidueiros, S. M., Curti, C. A., Pallaro, A. N., Bertero, H. D., et al. (2020). Oil quality in sea-level quinoa as determined by cultivar-specific responses to temperature and radiation conditions. J. Sci. Food Agri. 100, 1358–1361. doi: 10.1002/jsfa.10092
Curti, R. N., Sanahuja, M. D., Vidueiros, S. M., Pallaro, A. N., and Bertero, H. D. (2018). Trade-off between seed yield components and seed composition traits in sea level quinoa in response to sowing dates. Cereal Chem. 95, 734–741. doi: 10.1002/cche.10088
De Bock, P, Van Bockstaele, P., Muylle, F., Quataert, H., Vermeir, P., Eeckhout, P. M., et al. (2021b). yield and nutritional characterization of thirteen quinoa (Chenopodium quinoa Willd.) varieties grown in north-west Europe-part I. Plants 10, 689. doi: 10.3390/plants10122689
De Bock, P., Daelemans, P., Selis, L., Raes, L., Vermeir, K., Eeckhout, P. M., et al. (2021a). Comparison of the chemical and technological characteristics of wholemeal flours obtained from amaranth (Amaranthus sp.), quinoa (Chenopodium quinoa) and buckwheat (Fagopyrum sp.) seeds. Foods 10, 651. doi: 10.3390/foods10030651
Dong, J. L., Huang, L., Chen, W. W., Zhu, Y. Y., Dun, B. Q., Shen, R. L., et al. (2021). Effect of heat-moisture treatments on digestibility and physicochemical property of whole quinoa flour. Foods 10, 3042. doi: 10.3390/foods10123042
Dumschott, K., Wuyts, N., Alfaro, C., Castillo, D., Fiorani, F., Zurita-Silva, A., et al. (2022). Morphological and physiological traits associated with yield under reduced irrigation in chilean coastal lowland quinoa. Plants 11, 323. doi: 10.3390/plants11030323
El-Serafy, R. S., El-Sheshtawy, A. N. A., Abd El-Razek, U. A., Abd El-Hakim, A. F., Hasham, M. M. A., Sami, R. Al-Mushhin, A.a.M., et al. (2021). Growth, yield, quality, and phytochemical behavior of three cultivars of quinoa in response to moringa and azolla extracts under organic farming conditions. Agronomy 11, 186. doi: 10.3390/agronomy11112186
El-Sohaimy, S., and Mehany, T. (2015). Physicochemical and functional properties of quinoa protein isolate. Annal Agri. Sci. 60, 297–305. doi: 10.1016/j.aoas.2015.10.007
Encina-Zelada, C., Cadavez, V., Pereda, J., Gomez-Pando, L., Salva-Ruiz, B., Teixeira, J. A., et al. (2017). Estimation of composition of quinoa (Chenopodium quinoa Willd.) grains by near-infrared transmission spectroscopy. Lwt-Food Sci. Technol. 79, 126–134. doi: 10.1016/j.lwt.2017.01.026
Escuredo, O., Martin, M. I. G., Moncada, G. W., Fischer, S., and Hierro, J. M. H. (2014). Amino acid profile of the quinoa (Chenopodium quinoa Willd.) using near infrared spectroscopy and chemometric techniques. J. Cereal Sci. 60, 67–74. doi: 10.1016/j.jcs.2014.01.016
Eurisco (2022). The European Search Catalogue for Plant Genetic Resources (EURISCO). Available online at: https://eurisco.ipk-gatersleben.de/apex/f?p=10357::::P57_NATIONAL_INVENTORY,P57_INCLUDE_SYNONYMS:12,NO (accessed Februry 11, 2022).
Faostat (2022). Crops and livestock production. Rome, Italy: Statistics Division, Food and Agriculture Organization of the United Nations. Available online at: https://www.fao.org/faostat/en/#data/QCL (accessed January 31, 2022).
Ferreira, D. S. Pallone, J. A. L., and Poppi, R. J. (2015). Direct analysis of the main chemical constituents in Chenopodium quinoa grain using Fourier transform near-infrared spectroscopy. Food Control 48, 91–95. doi: 10.1016/j.foodcont.2014.04.016
Fischer, S., Wilckens, R., Jara, J., and Aranda, M. (2013). Variation in antioxidant capacity of quinoa (Chenopodium quinoa Will) subjected to drought stress. Ind. Crops Products 46, 341–349. doi: 10.1016/j.indcrop.2013.01.037
Galluzzi, G., and Noriega, I. L. (2014). Conservation and use of genetic resources of underutilized crops in the americas-a continental analysis. Sustainability 6, 980–1017. doi: 10.3390/su6020980
Garcia-Parra, M., Roa-Acosta, D., Garcia-Londono, V., Moreno-Medina, B., and Bravo-Gomez, J. (2021). Structural characterization and antioxidant capacity of quinoa cultivars using techniques of FT-MIR and UHPLC/ESI-Orbitrap MS spectroscopy. Plants 10, 159. doi: 10.3390/plants10102159
Garcia-Parra, M. A., Roa-Acosta, D. F., Bravo-Gomez, J. E., Hernandez-Criado, J. C., and Villada-Castillo, H. S. (2022). Effects of altitudinal gradient on physicochemical and rheological potential of quinoa cultivars. Front. Sust. Food Systems 6, 862238. doi: 10.3389/fsufs.2022.862238
Gardner, M., Maliro, M. F. A., Goldberger, J. R., and Murphy, K. M. (2019). Assessing the potential adoption of quinoa for human consumption in central Malawi. Front. Sust. Food Syst. 3, 52. doi: 10.3389/fsufs.2019.00052
Gargiulo, L., Grimberg, A., Repo-Carrasco-Valencia, R., Carlsson, A. S., and Mele, G. (2019). Morpho-densitometric traits for quinoa (Chenopodium quinoa Willd.) seed phenotyping by two X-ray micro-CT scanning approaches. J Cereal Sci. 90, 102829. doi: 10.1016/j.jcs.2019.102829
Genesys (2022). Available online at: https://www.genesys-pgr.org/a/v2Ok2159rW9 (accessed February 15, 2022).
Ghumman, A., Mudgal, S., Singh, N., Ranjan, B., Kaur, A., Rana, J. C., et al. (2021). Physicochemical, functional and structural characteristics of grains, flour and protein isolates of Indian quinoa lines. Food Res. Int. 140, 109982. doi: 10.1016/j.foodres.2020.109982
Gilani, G. S. Xiao, Ch. W., and Cockell, K. A. (2012). Impact og antinutritional factors in food proteins on the digestibility of protein and the bioavailability of amino acids and on protein quality. Br. J. Nutr. 108, S315–S332. doi: 10.1017/S0007114512002371
Gomez, M. J. R., Prieto, J. M., Sobrado, V. C., and Magro, P. C. (2021). Nutritional characterization of six quinoa (Chenopodium quinoa Willd) varieties cultivated in Southern Europe. J. Food Comp. Anal. 99, 103876. doi: 10.1016/j.jfca.2021.103876
Gonzalez, J. A., Konishi, Y., Bruno, M., Valoy, M., and Prado, F. E. (2012). Interrelationships among seed yield, total protein and amino acid composition of ten quinoa (Chenopodium quinoa) cultivars from two different agroecological regions. J. Sci. Food Agri. 92, 1222–1229. doi: 10.1002/jsfa.4686
Graf, B. L., Rojo, L. E., Delatorre-Herrera, J., Poulev, A., Calfio, C., Raskin, I., et al. (2016). Phytoecdysteroids and flavonoid glycosides among Chilean and commercial sources of Chenopodium quinoa: variation and correlation to physico-chemical characteristics. J. Sci. Food Agri. 96, 633–643. doi: 10.1002/jsfa.7134
Granado-Rodriguez, S., Aparicio, N., Matias, J., Perez-Romero, L. F., Maestro, I., Graces, I., et al. (2021a). Studying the impact of different field environmental conditions on seed quality of quinoa: the case of three different years changing seed nutritional traits in southern Europe. Front. Plant Sci. 12, 649132. doi: 10.3389/fpls.2021.649132
Granado-Rodriguez, S., Vilarino-Rodriguez, S., Maestro-Gaitan, I., Matias, J., Rodriguez, M. J., Calvo, P., et al. (2021b). Genotype-dependent variation of nutritional quality-related traits in quinoa seeds. Plants 10, 128. doi: 10.3390/plants10102128
Granda, L., Rosero, A., Benesova, K., Pluhackova, H., Neuwirthova, J., Cerkal, R., et al. (2018). Content of selected vitamins and antioxidants in colored and nonpigmented varieties of quinoa, barley, and wheat grains. J. Food Sci. 83, 2439–2447. doi: 10.1111/1750-3841.14334
Grimberg, A., Saripella, G. V., Valencia, R., Bengtsson, T., Alandia, G., Carlsson, A. S., et al. (2022). Transcriptional regulation of quinoa seed quality: identification of novel candidate genetic markers for increased protein content. Front. Plant Sci. 13, 816425. doi: 10.3389/fpls.2022.816425
Habiyaremye, C., Ndayiramije, O., Guedes, J. D., and Murphy, K. M. (2022). Assessing the adaptability of quinoa and millet in two agroecological zones of Rwanda. Front. Sust. Food Syst. 6, 850280. doi: 10.3389/fsufs.2022.850280
He, X., Wang, B., Zhao, B., and Yang, F. (2022). Ultrasonic assisted extraction of quinoa (Chenopodium quinoa Willd.) protein and effect of heat treatment on uts in vitro digestion characteristics. Foods 11, 771. doi: 10.3390/foods11050771
Hinojosa, L., Leguizamo, A., Carpio, C., Munoz, D., Mestanza, C., Ochoa, J., et al. (2021). Quinoa in ecuador: recent advances under global expansion. Plants 10, 298. doi: 10.3390/plants10020298
Hussain, M. I., Farooq, M., Syed, Q. A., Ishaq, A., Al-Ghamdi, A. A., Hatamleh, A. A., et al. (2021). Botany, nutritional value, phytochemical composition and biological activities of quinoa. Plants 10, 2258. doi: 10.3390/plants10112258
Hussain, M. I., Muscolo, A., Ahmed, M., Asghar, M. A., and Al-Dakheel, A. J. (2020). Agro-morphological, yield and quality traits and interrelationship with yield stability in Quinoa (Chenopodium quinoa Willd.) genotypes under saline marginal environment. Plants 9, 1763. doi: 10.3390/plants9121763
Jacobsen, S. E. (2011). The situation for quinoa and its production in southern bolivia: from economic success to environmental disaster. J. Agron. Crop Sci. 197, 390–399. doi: 10.1111/j.1439-037X.2011.00475.x
Jacobsen, S. E. (2017). The scope for adaptation of quinoa in Northern Latitudes of Europe. J. Agron. Crop Sci. 203, 603–613. doi: 10.1111/jac.12228
Jacobsen, S. E., and Christiansen, J. L. (2016). Some agronomic strategies for organic quinoa (Chenopodium quinoa Willd). J. Agron. Crop Sci. 202, 454–463. doi: 10.1111/jac.12174
Jacobsen, S. E., and Mujica, A. (2002). Genetic resources and breeding of the Andean grain crop quinoa (Chenopodium quinoa Willd). Plant Genetic Res. Newsletter 130, 54–61.
Jacobsen, S. E., Mujica, A., and Ortiz, R. (2003). La importancia de los cultivos andinos. Fermentum 13, 14–24.
Jiang, F., Ren, Y. J., Du, C. W., Nie, G., Liang, J. B., Yu, X. Z., et al. (2021). Effect of pearling on the physicochemical properties and antioxidant capacity of quinoa (Chenopodium quinoa Willd.) flour. J. Cereal Sci. 102, 103330. doi: 10.1016/j.jcs.2021.103330
Jimenez, M. D., Lobo, M., and Samman, N. (2019), 12th. IFDC 2017 Special Issue - Influence of germination of quinoa (Chenopodium quinoa) amaranth (Amaranthus) grains on nutritional techno-functional properties of their flours. J. Food Comp. Anal. 84, 103290. 10.1016/j.jfca.2019.103290
Kheto, A., Joseph, D., Islam, M., Dhua, S., Das, R., Kumar, Y., et al. (2022). Microwave roasting induced structural, morphological, antioxidant, and functional attributes of Quinoa (Chenopodium quinoa Willd). J. Food Proc. Preserv. 46, e16595. doi: 10.1111/jfpp.16595
Koziol, M. J. (1992). Chemical composition and nutritional value of quinoa (Chenopodium quinoa Willd). J. Food Comp. Anal. 5, 35–68. doi: 10.1016/0889-1575(92)90006-6
Lesjak, J., and Calderini, D. F. (2017). Increased night temperature negatively affects grain yield, biomass and grain number in chilean quinoa. Front. Plant Sci. 8, 352. doi: 10.3389/fpls.2017.00352
Li, G. T., Wang, S. N., and Zhu, F. (2016). Physicochemical properties of quinoa starch. Carbohydrate Polym. 137, 328–338. doi: 10.1016/j.carbpol.2015.10.064
Li, G. T., and Zhu, F. (2017). Molecular structure of quinoa starch. Carbohydrate Polym. 158, 124–132. doi: 10.1016/j.carbpol.2016.12.001
Lorusso, A., Verni, M., Montemurro, M., Coda, R., Gobetti, M., Rizzelo, C. G., et al. (2017). Use of fermented quinoa flour for pasta making and evaluation of the technological and nutritional features. LWT Food Sci. Technol. 78, 215–221. doi: 10.1016/j.lwt.2016.12.046
Manjarres-Hernandez, E. H., Arias-Moreno, D. M., Morillo-Coronado, A. C., Ojeda-Perez, Z. Z., and Cardenas-Chaparro, A. (2021). Phenotypic characterization of quinoa (Chenopodium quinoa Willd.) for the selection of promising materials for breeding programs. Plants 10, 1339. doi: 10.3390/plants10071339
Maradini, A. M., Pirozi, M. R., Borges, J. T. D. Sant'ana, H.M.P., Chaves, J.B.P., and Coimbra, J. (2017). Quinoa: Nutritional, functional, and antinutritional aspects. Crit. Rev. Food Sci. Nutr. 57, 1618–1630. doi: 10.1080/10408398.2014.1001811
Martin, M. I. G., Moncada, G. W., Fischer, S., and Escuredo, O. (2014). Chemical characteristics and mineral composition of quinoa by near-infrared spectroscopy. J. Sci. Food Agri. 94, 876–881. doi: 10.1002/jsfa.6325
Matías, J., Rodriguez, M. J., Cruz, V., Calvo, P., and Reguera, M. (2021). Heat stress lowers yields, alters nutrient uptake and changes seed quality in quinoa grown under Mediterranean field conditions. J. Agron. Crop Sci. 207, 481–491. doi: 10.1111/jac.12495
Matias, J., Rodriguez, M. J., Granado-Rodriguez, S., Cruz, V., Calvo, P., Reguera, M., et al. (2022). Changes in quinoa seed fatty acid profile under heat stress field conditions. Front. Nutr. 9, 820010. doi: 10.3389/fnut.2022.820010
Mhada, M., Metougui, M. L., El Hazzam, K., El Kacimi, K., and Yasri, A. (2020). Variations of saponins, minerals and total phenolic compounds due to processing and cooking of quinoa (Chenopodium quinoaWilld.) Seeds. Foods 9, 660. doi: 10.3390/foods9050660
Miranda, M., Vega-Galvez, A., Martinez, E., Lopez, J., Rodriguez, M. J., Henriquez, K., et al. (2012). Genetic diversity and comparison of physicochemical and nutritional characteristics of six quinoa (Chenopodium quinoa willd.) genotypes cultivated in Chile. Ciencia E Tecnol. De Alimentos 32, 835–843. doi: 10.1590/S0101-20612012005000114
Miranda, M., Vega-Galvez, A., Martinez, E. A., Lopez, J., Marin, R., Aranda, M., et al. (2013). Influence of contrasting environments on seed composition of two quinoa genotypes: nutritional and functional properties. Chilean J. Agric. Res. 73, 108–116. doi: 10.4067/S0718-58392013000200004
Mosyakin, S. L., and Schwartau, V. V. (2015). Quinoa as a promising pseudocereal crop for Ukraine. Agri. Sci. Prac. 2, 3–11. doi: 10.15407/agrisp2.01.003
Mota, C., Santos, M., Mauro, R., Samman, N., Matos, A. S., Torres, D., et al. (2016). Protein content and amino acids profile of pseudocereals. Food Chem. 193, 55–61. doi: 10.1016/j.foodchem.2014.11.043
Nascimento, A. C., Mota, C., Coelho, I., Gueirao, S., Santos, M., Matos, A. S., et al. (2014). Characterisation of nutrient profile of quinoa (Chenopodium quinoa); amaranth (Amaranthus caudatus), and purple corn (Zea mays L.) consumed in the North of Argentina: Proximates, minerals and trace elements. Food Chem. 148, 420–426. doi: 10.1016/j.foodchem.2013.09.155
Nowak, V., Du, J., and Charrondiere, U. R. (2016). Assessment of the nutritional composition of quinoa (Chenopodium quinoa Willd). Food Chem. 193, 47–54. doi: 10.1016/j.foodchem.2015.02.111
Nuñez De Arco, S. (2015). “Quinoa's calling,” in Quinoa Improvement and Sustainable Production, eds. K. M. Murphy and J. Matanguihan (Hoboken, NJ: John Wiley and Sons, Inc.), 211–226.
Opazo-Navarrete, M., Tagle Freire, D., Boom, R. M., and Janssen, A. E. M. (2019). The influence of starch and fibre on in vitro protein digestibility of dry fractionated quinoa seed (Riobamba variety). Food Biophys. 14, 49–59. doi: 10.1007/s11483-018-9556-1
Paucar-Menacho, L. M., Duenas, M., Penas, E., Frias, J., and Martinez-Villaluenga, C. (2018). Effect of dry heat puffing on nutritional composition, fatty acid, amino acid and phenolic profiles of pseudocereals grains. Polish J. Food Nut. Sci. 68, 289–297. doi: 10.1515/pjfns-2018-0005
Pellegrini, M., Lucas-Gonzales, R., Ricci, A., Fontecha, J., Fernandez-Lopez, J., Perez-Alvarez, J. A., et al. (2018). Chemical, fatty acid, polyphenolic profile, techno-functional and antioxidant properties of flours obtained from quinoa (Chenopodium quinoa Willd) seeds. Ind. Crops Prod. 111, 38–46. doi: 10.1016/j.indcrop.2017.10.006
Peng, M. J., Yin, L. S., Dong, J. L., Shen, R. L., and Zhu, Y. Y. (2022). Physicochemical characteristics and in vitro digestibility of starches from colored quinoa (Chenopodium quinoa) varieties. J. Food Sci. 87, 2147–2158. doi: 10.1111/1750-3841.16126
Pereira, E., Encina-Zelada, C., Barros, L., Gonzales-Barron, U., Cadavez, V., Ferreira, I., et al. (2019). Chemical and nutritional characterization of Chenopodium quinoa Willd (quinoa) grains: A good alternative to nutritious food. Food Chem. 280, 110–114. doi: 10.1016/j.foodchem.2018.12.068
Pinto, A. A., Fischer, S., Wilckens, R., Bustamante, L., and Berti, M. T. (2021). Production efficiency and total protein yield in quinoa grown under water stress. Agriculture 11, 1089. doi: 10.3390/agriculture11111089
Prado, F. E., Fernandez-Turiel, J. L., Tsarouchi, M., Psaras, G. K., and Gonzalez, J. A. (2014). Variation of seed mineral concentrations in seven quinoa cultivars grown in two agroecological sites. Cereal Chem. 91, 453–459. doi: 10.1094/CCHEM-08-13-0157-R
Prager, A., Munz, S., Nkebiwe, P. M., Mast, B., and Graeff-Honninger, S. (2018). Yield and quality characteristics of different quinoa (Chenopodium quinoa Willd.) cultivars grown under field conditions in southwestern Germany. Agronomy 8, 197. doi: 10.3390/agronomy8100197
Pulvento, C., Riccardi, M., Lavini, A., Iafelice, G., and Marconi, E. D'andria, R. (2012). Yield and quality characteristics of quinoa grown in open field under different saline and non-saline irrigation regimes. J. Agron. Crop Sci. 198, 254–263. doi: 10.1111/j.1439-037X.2012.00509.x
Rahut, D. B., Aryal, J. P., Manchanda, N., and Sonobe, T. (2022). “Expectations for household food security in the coming decades: A global scenario,” in Future Foods. Global Trends, Opportunities, and Sustainability Challenges, ed. R. Bhat. (London: Academic Press), 107–131.
Reguera, M., Conesa, C. M., Gil-Gomez, A., Haros, C. M., Perez-Casa, M. A., Briones-Labarca, V., et al. (2018). The impact of different agroecological conditions on the nutritional composition of quinoa seeds. PEERJ 6, e4442. doi: 10.7717/peerj.4442
Repo-Carrasco, R., Espinoza, C., and Jacobsen, S. E. (2003). Nutritional value and use of the Andean crops quinoa (Chenopodium quinoa) and kaniwa (Chenopodium pallidicaule). Food Rev. Int. 19, 179–189. doi: 10.1081/FRI-120018884
Rizzello, C. G., Lorusso, A., Montemurro, M., and Gobbetti, M. (2016). Use of sourdough made with quinoa (Chenopodium quinoa) flour and autochthonous selected lactic acid bacteria for enhancing the nutritional, textural and sensory features of white bread. Food Microbiol. 56, 1–13. doi: 10.1016/j.fm.2015.11.018
Rojas, W., Milton, P., Alanoca, C., Gómez Pando, L., Leónlobos, P., Alercia, A., et al. (2015). “Quinoa genetic resources and ex situ conservation,” in State of the Art Report on Quinoa Around the World in 2013, eds. D. Bazile, D. Bertero and C. Nieto (Rome: FAO/CIRADE), 56–82.
Ruales, J., and Nair, B. M. (1992). Nutritional quality of the protein in quinoa (Chenopodium quinoa Willd.) seeds. Plant Foods Hum. Nutr. 42, 1–11. doi: 10.1007/BF02196067
Ruiz, K. B., Aloisi, I., Del Duca, S., Canelo, V., Torrigiani, P., Silva, H., et al. (2016). Salares versus coastal ecotypes of quinoa: salinity responses in Chilean landraces from contrasting habitats. Plant Physiol. Biochem. 101, 1–13. doi: 10.1016/j.plaphy.2016.01.010
Ruiz, K. B., Biondi, S., Oses, R., Acuna-Rodriguez, I. S., Antognoni, F., Martinez-Mosqueira, E. A., et al. (2014). Quinoa biodiversity and sustainability for food security under climate change. A review. Agron. Sust. Dev. 34, 349–359. doi: 10.1007/s13593-013-0195-0
Saad-Allah, K. M., and Youssef, M. S. (2018). Phytochemical and genetic characterization of five quinoa (Chenopodium quinoa Willd.) genotypes introduced to Egypt. Physiol. Mol. Biol. Plants 24, 617–629. doi: 10.1007/s12298-018-0541-4
Sanchez-Resendiz, A. I., Escalante-Aburto, A., Andia-Ayme, V., and Chuck-Hernandez, C. (2019). Structural properties, functional evaluation, and in vitro protein digestibility of black and yellow quinoa (Chenopodium petiolare) protein isolates. Cyta-J. Food 17, 864–872. doi: 10.1080/19476337.2019.1669714
Schlick, G., and Bubenheim, D. L. (1996). “Quinoa: candidate crop for NASA's controlled ecological life support systems,” in Progress in New Crops, ed. J. Janick (Arlington: ASHS Press), 632–640.
Schmidt, D., Verruma-Bernardi, M. R., Forti, V. A., and Borges, M. (2021). Quinoa and amaranth as functional foods: a review. Food Rev. Int. 37, 1–20. doi: 10.1080/87559129.2021.1950175
Shen, Y. B., Zheng, L. Y., Peng, Y., Zhu, X. C., Liu, F., Yang, X. Q., et al. (2022). Physicochemical, antioxidant and anticancer characteristics of seed oil from three Chenopodium quinoa genotypes. Molecules 27, 2453. doi: 10.3390/molecules27082453
Shi, D., Fidelis, M., Ren, Y., Stone, A. K., Ai, Y., Nickerson, M. T., et al. (2020). The functional attributes of Peruvian (Kankolla and Blanca juli blend) and Northern quinoa (NQ94PT) flours and protein isolates, and their protein quality. Food Res. Int. 128, 108799. doi: 10.1016/j.foodres.2019.108799
Simopoulos, A. P. (2002). The importance of the ratio of Omega-6/Omega-3 essential fatty acids. Biomed. Pharmacother. 56, 365–379. doi: 10.1016/S0753-3322(02)00253-6
Singh, M. P., Soni, K., Bhamra, R., and Mittal, R. K. (2022). Superfood: value and need. Curr. Nutr. Food Sci. 18, 65–68. doi: 10.2174/1573401317666210420123013
Sobota, A., Swieca, M., Gesinski, K., Wirkijowska, A., and Bochnak, J. (2020). Yellow-coated quinoa (Chenopodium quinoa Willd) – physicochemical, nutritional, and antioxidant properties. J. Sci. Food Agri. 100, 2035–2042. doi: 10.1002/jsfa.10222
Steffolani, M. E., Villacorta, P., Morales-Soriano, E. R., Repo-Carrasco, R., Leon, A. E., Perez, G. T., et al. (2016). Physicochemical and functional characterization of protein isolated from different quinoa varieties (Chenopodium quinoa Willd). Cereal Chem. 93, 275–281. doi: 10.1094/CCHEM-04-15-0083-R
Sun, Y., Liu, F., Bendevis, M., Shabala, S., and Jacobsen, S. E. (2014). Sensitivity of two quinoa (Chenopodium quinoaWilld.) varieties to progressive drought stress. J. Agron. Crop Sci. 200, 12–23. doi: 10.1111/jac.12042
Taaime, N., El Mejahed, K., Moussafir, M., Bouabid, R., Oukarroum, A., Choukr-Allah, R., et al. (2022). Early sowing of quinoa cultivars, benefits from rainy season and enhances quinoa development, growth, and yield under arid condition in morocco. Sustainability 14, 4010. doi: 10.3390/su14074010
Tabatabaei, I., Alseekh, S., Shahid, M., Leniak, E., Wagner, M., Mahmoudi, H., et al. (2022). The diversity of quinoa morphological traits and seed metabolic composition. Sci. Data 9, 1–7. doi: 10.1038/s41597-022-01399-y
Tang, Y., Li, X. H., Chen, P. X., Zhang, B., Hernandez, M., Zhang, H., et al. (2015). Characterisation of fatty acid, carotenoid, tocopherol/tocotrienol compositions and antioxidant activities in seeds of three Chenopodium quinoa Willd. genotypes. Food Chem. 174, 502–508. doi: 10.1016/j.foodchem.2014.11.040
Tang, Y., Li, X. H., Chen, P. X., Zhang, B., Liu, R. H., Hernandez, M., et al. (2016). Assessing the fatty acid, carotenoid, and tocopherol compositions of amaranth and quinoa seeds grown in Ontario and their overall contribution to nutritional quality. J. Agri. Food Chem. 64, 1103–1110. doi: 10.1021/acs.jafc.5b05414
Thiam, E., Allaoui, A., and Benlhabib, O. (2021). Quinoa productivity and stability evaluation through varietal and environmental interaction. Plants 10, 714. doi: 10.3390/plants10040714
Toderich, K. N., Mamadrahimov, A. A., Khaitov, B. B., Karimov, A. A., Soliev, A. A., Nanduri, K. R., et al. (2020). Differential impact of salinity stress on seeds minerals, storage proteins, fatty acids, and squalene composition of new quinoa genotype, grown in hyper-arid desert environments. Front. Plant Sci. 11, 607102. doi: 10.3389/fpls.2020.607102
Tovar, J. C., Quillatupa, C., Callen, S. T., Castillo, S. E., Pearson, P., Shamin, A., et al. (2020). Heating quinoa shoots results in yield loss by inhibiting fruit production and delaying maturity. Plant J. 102, 1058–1073. doi: 10.1111/tpj.14699
Tschopp, M., Bieri, S., and Rist, S. (2018). Quinoa and production rules: how are cooperatives contributing to governance of natural resources? In. J. Commons 12, 402–427. doi: 10.18352/ijc.826
USDA (2020). U.S. Department Of Agriculture Agricultural Research Service. Food Data Central. Available Online at: https://fdc.nal.usda.gov/ (accessed July 7, 2022).
Vera, E. P., Alca, J. J., Saravia, G. R., Campioni, N. C., and Alpuy, I. J. (2019). Comparison of the lipid profile and tocopherol content of four Peruvian quinoa (Chenopodium quinoa Willd.) cultivars (“Amarilla de Maranganf”, “Blanca de Juli”, INIA 415 “Roja Pasankalla”, INIA 420 “Negra Collana”) during germination. J. Cereal Sci. 88, 132–137. doi: 10.1016/j.jcs.2019.05.015
Vidueiros, S. M., Curti, R. N., Dyner, L. M., Binaghi, M. J., Peterson, G., Bertero, H. D., et al. (2015). Diversity and interrelationships in nutritional traits in cultivated quinoa (Chenopodium quinoa Willd.) from Northwest Argentina. J. Cereal Sci. 62, 87–93. doi: 10.1016/j.jcs.2015.01.001
Villacres, E., Quelal, M., Galarza, S., Iza, D., and Silva, E. (2022). Nutritional value and bioactive compounds of leaves and grains from quinoa (Chenopodium quinoa Willd). Plants 11, 213. doi: 10.3390/plants11020213
Walters, H., Carpenter-Boggs, L., Desta, K., Yan, L., Matanguihan, J., Murphy, K., et al. (2016). Effect of irrigation, intercrop, and cultivar on agronomic and nutritional characteristics of quinoa. Agroecol. Sust. Food Syst. 40, 783–803. doi: 10.1080/21683565.2016.1177805
Wang, N., Wang, F. X., Shock, C. C., Meng, C. B., and Qiao, L. F. (2020). Effects of management practices on quinoa growth, seed yield, and quality. Agronomy 10, 445. doi: 10.3390/agronomy10030445
WHO/FAO/UNU (2007). Protein and amino acid requirements in human nutrition report of a joint WHO/FAO/UNU expert consultation (Geneva: World Health Organization).
Wu, G. Y., Peterson, A. J., Morris, C. F., and Murphy, K. M. (2016). Quinoa seed quality response to sodium chloride and sodium sulfate salinity. Front. Plant Sci. 7, 790. doi: 10.3389/fpls.2016.00790
Wu, L. G., Wang, A. N., Shen, R. L., and Qu, L. B. (2020). Effect of processing on the contents of amino acids and fatty acids, and glucose release from the starch of quinoa. Food Sci. Nutr. 8, 4877–4887. doi: 10.1002/fsn3.1775
Keywords: amino acids, genetic resources, nutritive value, protein, quinoa, environmental conditions
Citation: Hlásná Cepková P, Dostalíková L, Viehmannová I, Jágr M and Janovská D (2022) Diversity of quinoa genetic resources for sustainable production: A survey on nutritive characteristics as influenced by environmental conditions. Front. Sustain. Food Syst. 6:960159. doi: 10.3389/fsufs.2022.960159
Received: 02 June 2022; Accepted: 10 October 2022;
Published: 26 October 2022.
Edited by:
Ivan Kreft, University of Ljubljana, SloveniaReviewed by:
Sapna Langyan, National Bureau of Plant Genetic Resources (ICAR), IndiaMateja Germ, University of Ljubljana, Slovenia
Copyright © 2022 Hlásná Cepková, Dostalíková, Viehmannová, Jágr and Janovská. This is an open-access article distributed under the terms of the Creative Commons Attribution License (CC BY). The use, distribution or reproduction in other forums is permitted, provided the original author(s) and the copyright owner(s) are credited and that the original publication in this journal is cited, in accordance with accepted academic practice. No use, distribution or reproduction is permitted which does not comply with these terms.
*Correspondence: Dagmar Janovská, janovska@vurv.cz