Mixed FSO/RF Based Multiple HAPs Assisted Multiuser Multiantenna Terrestrial Communication
- Computer, Electrical, and Mathematical Science and Engineering (CEMSE) Division, King Abdullah University of Science and Technology (KAUST), Thuwal, Saudi Arabia
In this work, a mixed free-space optics (FSO)/radio-frequency (RF) based multiple serial high altitude platforms (HAPs) assisted multiuser multiantenna terrestrial communication system is considered. For the considered multi-hop system, earth station to HAP and HAP to HAP links are assumed as FSO links, and HAP to terrestrial mobile users (MUs) link is assumed as RF link. At the FSO detector both the heterodyne detection and intensity modulation direct detection techniques are considered. Atmospheric turbulence of the ES to HAP FSO link is modeled with Gamma-Gamma fading along with the pointing error impairments. As HAP situates in the stratosphere, negligible atmospheric turbulence exists at HAP altitude (20 km), hence, only pointing error is considered for the inter HAP links. Further, the HAP consists of multiantenna array to provide high data-rates to the terrestrial users via RF links, considered to be Nakagami-m distributed. The intermediate HAPs perform selective decode-and-forward relaying and opportunistic user scheduling is performed for the best user selection. For the performance analysis, analytical expression of overall outage probability is obtained and the impact of various selection parameters like pointing error, FSO detection type, MU selection, number of antennas, and RF fading severity are observed on the outage performance. Asymptotic outage probability is also derived to obtain the diversity order of the considered communication system. Further, considering various modulation schemes, a generalized average bit-error-rate expression is derived and the impact of pointing error, FSO detection type, MU selection, number of antennas, and RF fading severity are observed on its performance. Finally, derived results are validated through Monte-Carlo simulations.
1 Introduction
For reliable and robust high data-rate communication for various wireless applications including the emergency disastrous situations, temporary mass events, and even in military applications, promptly deployable flying base stations such as unmanned aerial vehicles (UAVs) have gained significant research attention as they are capable to provide high data-rates with improved capacity and coverage, targeted for beyond fifth generation (5G) communications(Zhan et al., 2011; Mozaffari et al., 2019). UAVs are broadly categorized into low altitude platform (LAP) and high altitude platform (HAP) based on their size and operating range. The LAPs are situated only few hundred meters above the earth surface, however, the HAPs are usually situated in stratosphere around 17–25 km (km) altitude from the earth surface. As the HAP situates in stratosphere, the wind velocity is low and atmospheric turbulence is negligible, hence, the HAP can be in quasi-static position and is capable to provide ubiquitous connectivity (Kurt et al., 2021). Due to the enhancement in communications technologies, lightweight composite materials, improved efficiency of solar panels and antenna, HAP became an viable aerial network component for various communication applications. The HAPs can be deployed easily and rapidly, their maintenance is easy, and having moderate operational cost than satellite which makes them economically feasible (Fidler et al., 2010; Kurt et al., 2021). Various technological trends of HAPs and their applications in wireless communications have been seen in (d’Oliveira et al., 2016). On the other hand, free-space optics (FSO) has been proven as an efficient replacement to radio-frequency (RF) due to its high operational bandwidth with improved capacity to fulfill the increasing demand of high data-rates for present and future wireless communication networks. FSO operates in unlicensed band, having low cost and easy deployment, and provides high-speed line-of-sight (LoS) communication (Singya et al., 2020; Trichili et al., 2020). The FSO links provide high data-rate secured transmission than the RF links, however requires LoS communication. Further, atmospheric turbulence and weather issues are more severe in terrestrial mobile communication which limits the FSO link’s performance. In such situations, RF links provide more robust communication to mobile users (MUs) than the FSO links. Thus, a hybrid system which can utilize the high data-rate capability of FSO links and the reliable transmission through RF links to terrestrial MUs has gained significant research attention (Trichili et al., 2021).
Various HAP networks like HAPCOS and CAPANINA in Europe, Helicos in USA, SkyNet is Asia, etc. have been deployed successfully for broadband data transmission to a larger distance (in hundreds of km). A considerable work on HAP networks have been seen in the literature. In (Vaiopoulos et al., 2013), authors proposed a HAP assisted setup for WiMAX orthogonal frequency division multiplexing (OFDM) transmission and derived the outage probability. In (Fidler et al., 2010), authors have shown various field trials to and from HAP using optical links to provide high data-rates. In (David et al., 2004), various considerations for inter-HAP optical link design are shown. In (Parthasarathy et al., 2014), channel modeling of inter-HAP optical link is proposed. In (Shibata et al., 2020), a gigabit HAP system for mobile communications is proposed. An HAP assisted ground to satellite uplink FSO/RF system is proposed in (Swaminathan et al., 2021) with Gamma-Gamma and shadowed-Rician fading for FSO and RF links, respectively, and various performance measures are derived. In (Singya and Alouini, 2021), an HAP assisted downlink hybrid FSO/RF based multiuser multiantenna terrestrial communication system is proposed, where the FSO link is characterized with the Gamma-Gamma distribution with pointing error impairments and Nakagami-m distribution is preferred for RF links. For the performance analysis, analytical expressions of outage probability, asymptotic outage probability, ergodic capacity, effective capacity, and generalized average symbol-error-rate (ASER) expressions of hexagonal-quadrature amplitude modulation (QAM), cross-QAM, and rectangular QAM are derived. (Liu et al., 2020) focuses on amplify-and-forward (AF) based HAP assisted satellite-terrestrial communication with Gamma-Gamma and Rayleigh distributions for the FSO and RF links, respectively, and obtained the outage probability. In (Antonini et al., 2006), capacity and efficiency of FSO link between the satellite and HAP are obtained for its feasibility. In (Gao et al., 2021), optimization of an intelligent reconfigurable surface assisted HAP network is proposed for downlink communication to terrestrial MUs.
However, a significant work is witnessed on various hybrid FSO/RF systems in the literature. (Bhatnagar and Arti, 2013) considers a hybrid RF/FSO based satellite-terrestrial communication system, where Gamma-Gamma and shadowed-Rician distributions are considered for the FSO and RF links, respectively. Further, analytical ASER expression for M-ary phase-shift keying (MPSK) and asymptotic results are obtained. (Zedini et al., 2016) focuses on a hybrid FSO/RF system with Nakagami-m and Gamma-Gamma fading for the RF and FSO links, respectively, and the analytical expressions of ergodic capacity, outage probability, and bit-error-rate (BER) of binary phase-shift keying (BPSK) are derived. (Chen et al., 2019) covers energy harvesting in hybrid FSO/RF system by obtaining the outage probability. An FSO/RF based satellite-terrestrial communication system is considered in (Ahmad et al., 2017), where satellite is connected through the optical feeder link and provides RF connectivity to terrestrial users, and various performance measures are derived for analysis. (Zedini et al., 2020) focuses on an FSO/RF based satellite-terrestrial communication system with Gamma-Gamma distributed uplink optical feeder link and shadowed-Rician distributed downlink RF terrestrial users links, and ergodic capacity, outage probability, and BER expressions of BPSK are derived. In (Sharma et al., 2019), switching-based FSO/RF system is proposed and various performance measures are obtained. (Lee et al., 2020) focuses on the data throughput maximization for UAV based FSO/RF system. Further, in (Singya et al., 2020), ASER performance of various higher order complex QAM schemes is obtained for a mixed RF/FSO system with outdated channel state information. In (Xu and Song, 2021) and (Xu and Song, 2020), hybrid RF/FSO based communication systems are considered, where RF link is characterized with κ − μ distribution and the FSO link is characterized with the
In the literature, some papers have discussed FSO based multi-hop communication systems. In (Datsikas et al., 2010; Zedini and Alouini, 2015; Ashrafzadeh et al., 2020), authors have analyzed the performance of multi-hop FSO systems. (Altubaishi and Alhamawi, 2019) focuses on the capacity analysis of a multi-hop FSO/RF system. In (Wang et al., 2015), authors have discussed the performance of multi-hop exponentiated Weibull distributed FSO system. However, above discussed multi-hop works focus only on generalized FSO systems without including HAP networks.
On the other hand, HAPs are having a wide 500 km radius footprint as recommended by International Telecommunication Union (ITU) (ITU-Radiocommun, 2000). Further, most of the HAP assisted projects have smaller coverage area and combining multiple HAPs can provide coverage to even entire country. For example, authors in (Miura and Oodo, 2001) and (Milas et al., 2003) have proposed respectively 16 and 18 HAPs configurations to provide coverage to entire Japan and Greece. However, only few works focus on the performance of multi-hop HAP/LAP assisted communication system. In (Yang et al., 2018), outage probability of LAP assisted RF/FSO/RF multi-hop system is derived. In (Sharma et al., 2016), authors have evaluated the performance of inter-HAP FSO communication system. Authors in (Michailidis et al., 2018) have discussed the performance of a three-hop HAP assisted RF/FSO/RF communication system. The above discussed works are summarized in Table 1 in details. The various considerations, channel models, and derived performance metrics are shown in Table 1 which are compared with our work (highlighted in bold letters) to show the novelty of our work.
As discussed above, we have seen significant works on dual-hop hybrid FSO/RF based systems. However, very limited work is seen on HAP assisted multi-hop hybrid FSO/RF scenario. Motivated with this, in this work, a highly reliable, robust, and high capacity long distance multiple serial HAPs assisted mixed FSO/RF multiuser multiantenna communication system is proposed. In the proposed system, end-to-end (e2e) long distance communication is completed through high bandwidth, high capacity FSO links established between the earth station (ES) to HAP and between multiple serial HAPs situated several hundred kilometer apart, and finally to the terrestrial MUs through the RF links via multiantenna array deployed at the final HAP. The intermediate HAPs are performing selective decode-and-forward (DF) relaying. Atmospheric turbulence of the ES to HAP link is modeled with Gamma-Gamma fading along with the pointing error impairments. As HAP situates at 20 km altitude, negligible atmospheric turbulence exists hence, only pointing error is considered for the inter HAP links. A multiantenna array is deployed at the HAP and Nakagami-m fading is considered for the RF links. Further, opportunistic user scheduling is performed for the best user selection. From this prospective, the major contributions of this work are as follows:
• For the performance analysis, individual link’s outage probability and overall outage probability are derived and the impact of pointing error, FSO detection type, MU selection, number of antennas, and RF fading severity are observed on the outage performance.
• To obtain the diversity order of the communication system, asymptotic outage probability is derived by performing the high SNR approximation.
• Considering various modulation schemes, a generalized average BER (ABER) expression is also derived and the impact of pointing error, FSO detection type, MU selection, number of antennas, and RF fading severity are observed on its performance.
Note that the proposed system model and the analysis shown in this work is generalized. By considering number of HAPs N = 1, the multi-hop serial HAPs assisted mixed FSO/RF system can be converted into a dual-hop HAP assisted mixed FSO/RF system. By considering number of users U = 1, the performance of Uth terrestrial MU can be obtained. Further, all the parameters can be adjusted and the considered system model can be deployed for various specific applications.
Rest of the work is organized as follows: In Section II, proposed system model and channel model are discussed in detail. Various performance measures like outage probability, asymptotic outage probability, and generalized ABER are discussed in detail in Section III, Section IV, and Section V, respectively. Section VI discusses various theoretical and simulation results of the proposed system and conclusions from the obtained results are finally illustrated in Section VII.
2 System and Channel Models
2.1 System Model
In this work, a mixed FSO/RF based multiuser multiantenna inter-HAP configuration is considered to provide high data-rates to the terrestrial MUs as shown in Figure 1. We have considered a communication scenario, where a source ES is communicating to the terrestrial MUs which are far apart through the use of multiple serial HAPs. Initially, the ES transmits the optical signal s(t) to HAP in first time slot. Hence, the received optical signal at the first HAP can be expressed as
where η is the optical-to-electrical conversion coefficient and r decides the type of FSO detector, wherein r = 1 corresponds to the heterodyne detection and r = 2 represents the IM/DD. Here I = IaIp represents the fading coefficient of the FSO link, where Ia is the fading due to atmospheric turbulence and Ip represents the pointing error associated with the FSO link. Further,
N intermediate HAPs with DF relaying are considered for e2e signal transmission. Hence, at the HAP, the received signal is first decoded, re-encoded, and then transmitted to the next HAP. The received signal can only be decoded successfully if the instantaneous received SNR at nth HAP is greater than or equal to the threshold SNR i.e.
Let,
where
Finally, the decoded signal from the Nth HAP is transmitted to the terrestrial MUs. To improve the channel capacity of the RF link and to improve its reliability, Nt transmit antennas are deployed at the HAP. However, due to size limit constraint, only single antenna is assumed at the terrestrial MUs. Hence, in the last-hop, the signal received from the Nth HAP at the uth MU after transmit beamforming is given as
where PN is the Nth HAP’s transmit power and
where GtH is the Nth HAP’s transmitter gain, Gru is the uth user’s receiver gain, LF = 20 log10fRF + 20 log10Lu + 92.45 is the free-space loss of the RF link between the HAP and uth terrestrial user of Lu distance (in km) with fRF transmission frequency, LA is the gaseous atmospheric loss, LR is the loss by rain attenuation in dB/km, and LO represents the miscellaneous losses including antenna degradation, polarization mismatch, antenna mispointing, etc. Hence, e2e instantaneous received SNR at the uth MU is calculated as
2.2 Channel Model
2.2.1 Earth Station to HAP Link
ES-HAP link is considered as an FSO link and its fading coefficient is characterized as I = IpIa, where Ip is the pointing error and Ia is the atmospheric turbulence induced fading. Let us assume, a Gaussian beam of beamwidth ωL is propagating L distance from the transmitter to receiver with a aperture radius, the fraction of the transmitted power collected by the receiver is approximately given as (Farid and Hranilovic, 2007)
where A0 represents the fraction of the collected power at rd = 0, rd is the radial displacement between the detector and beam center, and
Considering the case of identical jitters and zero boresight error, mean values ψx and ψy of the radial vectors dx and dy are zero and variances are identical as
where
The atmospheric turbulence Ia can be modeled with Gamma-Gamma distribution, its probability density function (PDF) is given as
where Kv(⋅) represents the vth order modified Bessel function of second kind. Further, α and β are the fading coefficients of the atmospheric turbulence which are given as
where
where θ is the zenith angle, k0 = 2π/λo denotes the wave number, H is the HAP altitude, and h0 is the ES height. Further,
where
Considering the identical jitters and zero boresight error case, the resulting conditional distribution is expressed as
Substituting (10) and (15) in (14), we get
Representing the modified Bessel function of the second kind into Meijer-G form as
Instantaneous received SNR of the ES-HAP FSO link is calculated as γH = μr(I)r, where r represents the type of FSO detector and μr is the average power of the FSO link. For heterodyne detection, r = 1, and hence,
Substituting (18) in
where
2.2.2 Inter HAP Links
The HAP is situated in the stratosphere above the clouds and there is almost negligible atmospheric turbulence. Hence, only the pointing error is considered between inter-HAP links. As identical jitters with zero boresight error is considered, the pointing error can be modeled by Rayleigh distribution and its PDF is given in (9). Now instantaneous received SNR at the nth HAP for the FSO link is calculated as
Performing
2.2.3 HAP to MUs RF Links
To connect the terrestrial MUs, communication between the HAP and the MUs is established through the RF links. Considering the size constraint of the MUs, single antenna is assumed at the MUs. Further, multiple transmit antennas (Nt) are considered at the HAP to avail the antenna diversity. The Nakagami-m distribution is considered for the RF link i.e. Nak(m, Ω), where m is the fading severity and Ω is the average power of the RF link. Hence, the CDF and PDF of the uth MU’s SNR can be given as
respectively, where Γ(⋅) represents the complete gamma function and
Invoking the PDF and CDF values from (22) in (23) and using the series representation of the incomplete Gamma function with multinomial expansion using (Gradshteyn and Ryzhik, 2000, (0.314)), the PDF of γHM can be derived as
where
where
3 Outage Probability
Outage probability is one of the important performance measures and defines the probability of reaching the instantaneous e2e SNR below a fixed threshold (γth). For the considered system, e2e instantaneous received SNR is shown in (7), hence the overall outage probability is given as
Substituting the respective CDF values of the individual links in (26), we get
Using the binomial series expansion and after some mathematical computations, (27) can be solved as
4 Asymptotic Outage Probability
Various insights on the system’s performance can be highlighted through the obtained outage probability (28). However, (28) is quite complex to decide the diversity order of the considered system. Hence, in this Section, we have conducted asymptotic analysis on the outage probability to obtain the diversity order. For this, the transmit SNR is assumed to tend to infinity. Therefore, at high SNR, (26) can be approximated as
Corollary 1: By using the identities as shown in (34) and (35), asymptotic outage probability for the considered system is expressed as
From (34), we observed that
Proof: See the Appendix.
5 Average BER Analysis
For various modulation schemes, a generalized ABER expression for the considered communication system can be given as (Zedini et al., 2020)
where n0, κ, and gp are the selection parameters for various modulation schemes and their values are shown in Table 2.
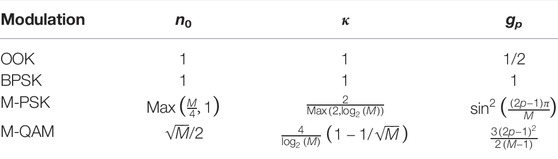
TABLE 2. Selection parameters for various modulation schemes (Zedini et al., 2020).
Please note that by using the IM/DD, we can obtain the ABER performance of on-off keying (OOK), preferred commonly in practical FSO systems as it is flexible to laser nonlinearity with ease of use. Further, we have analyzed the ABER performance of various MPSK (including the BPSK) and MQAM schemes using the coherent heterodyne detection at FSO receivers. Now plugin (28) in (31), and performing various mathematical computations by using (Gradshteyn and Ryzhik, 2000, (3.351),(6.455),(7.813), (8.352)) to solve the integrals, the final generalized analytical ABER expression is derived as
where
wherein
6 Theoretical and Simulation Results
In this Section, numerical values from the derived results are obtained and are validated through the simulations performed through Matlab by conducting 108 realizations. The atmospheric turbulence of ES-HAP link is generated by the product of two generalized Gamma distributed random variables. The radial displacement parameter is considered to be Rayleigh distributed and is applied to (8) for pointing error impairments. Throughout the analysis, η = 1 is considered. Unity transmit power is considered at all the nodes and γth = 1 dB is considered for the analysis. Further, parameters related to various links are shown in Table 3 unless otherwise stated.
In Figure 3, analytical and simulation results of outage probability against transmit SNR are shown for all three links. In Figure 3A, analytical and simulation results of ES-HAP link are compared for both the IM/DD and heterodyne detection (Het. in figures) techniques for different atmospheric turbulence conditions based on various ground turbulence i.e.
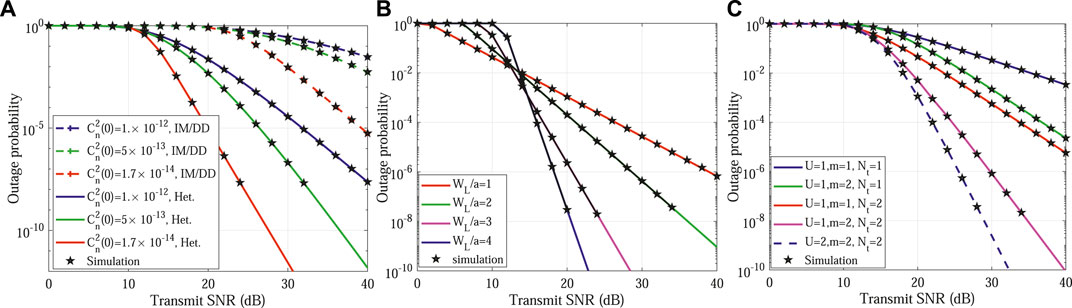
FIGURE 3. Comparison of analytical and simulation results of individual link’s outage probability against transmit SNR for (A) ES-HAP link, (B) HAP-HAP link, and (C) HAP-MUs link.
In Figure 4, analytical, simulation, and asymptotic results of overall system outage probability are compared for various values of U, m, and Nt while heterodyne detection is considered at FSO receiver. For the analysis,
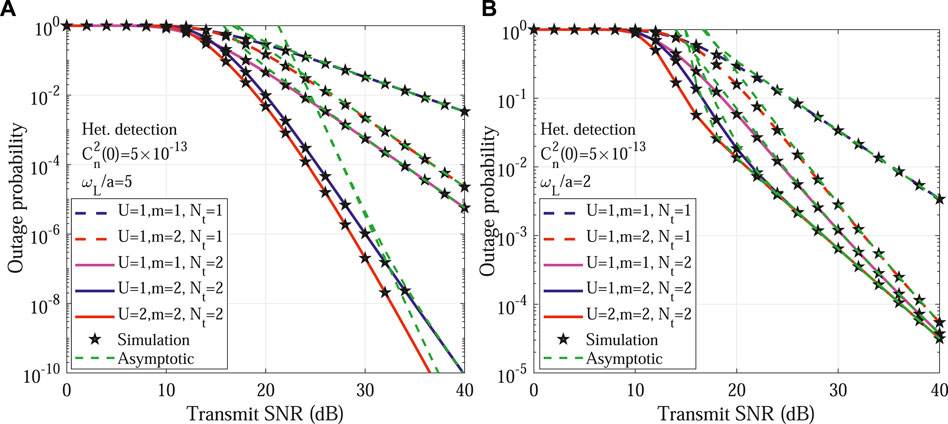
FIGURE 4. Analytical, simulation, and asymptotic results of overall outage probability against transmit SNR for (A) Moderate pointing error and (B) Severe pointing error.
In Figure 5, analytical and simulation results of ABER for 4-QAM are compared for various values of U, m, and Nt while heterodyne detection is considered at the FSO receiver. For the analysis,
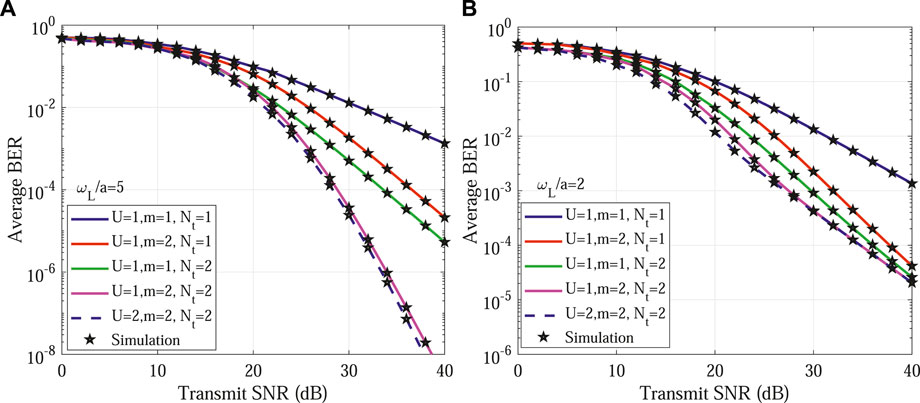
FIGURE 5. Analytical and simulation results of ABER against transmit SNR for 4-QAM with heterodyne detection at FSO receiver for (A) Moderate pointing error and (B) Severe pointing error.
In Figure 6, ABER of various modulation schemes are compared against transmit SNR. For OOK modulation, IM/DD detection is considered at the FSO receiver while heterodyne detection is preferred for the rest of the complex modulation schemes. For the analysis, U = 1, m = 1, and Nt = 2 are considered and other parameters are mentioned in Table 3. It is observed that BPSK provides significant gain in ABER performance than OOK as heterodyne detection provides significant performance improvement over IM/DD. To achieve an ABER of 10–2, BPSK provides around 15 dB gain over the OOK. Further, it is observed that the ABER performance of 4-QAM is same as 4-PSK. However, with the increase in constellation order, MQAM outperforms the MPSK. For an ABER of 10–3, 16-QAM provides approximately 3.2 dB gain over the 16-PSK. This gain improves further with the increase in M.
7 Conclusion
In this work, a mixed FSO/RF based multiple serial HAPs assisted multiuser multiantenna terrestrial communication system was considered, where ES-HAP and HAP-HAP links were FSO links and HAP to terrestrial MUs link was an RF link. Both the heterodyne detection and IM/DD techniques were considered at FSO receivers. The atmospheric turbulence of ES-HAP link was modeled with gamma-gamma fading with pointing error impairments. The HAP-HAP links were assumed to have pointing errors only. Multiantenna array was considered at the Nth HAP and best user selection was performed to improve the performance of RF links for the terrestrial users which were Nakagami-m distributed. For the performance analysis, analytical expressions of individual link’s outage probability, overall outage probability, asymptotic outage probability, and a generalized ABER expression of various modulation schemes were derived. Finally, the impact of various selection parameters like pointing error, FSO detection type, MU selection, RF fading severity, and the number of antennas were observed on their performance. The proposed system model and the analysis shown in this work is generalized. All the parameters can be adjusted and the considered system model can be deployed for various specific wireless communication applications. By considering number of HAPs equal to one, the multi-hop serial HAPs assisted hybrid FSO/RF system can be converted into a dual-hop HAP assisted hybrid FSO/RF system which can be applied for a short range highly reliable and robust high data-rate communication for various wireless applications including the emergency disastrous situations, temporary mass events, and even in military applications. By considering N HAPs in a serial mode, the coverage for the communication can be improved till several hundred kilometers and the problem of connecting the unconnected can be solved.
Data Availability Statement
The original contributions presented in the study are included in the article/Supplementary Material, further inquiries can be directed to the corresponding author.
Author Contributions
All authors listed have made a substantial, direct, and intellectual contribution to the work and approved it for publication.
Funding
This work is supported by the King Abdullah University of Science and Technology (KAUST), Saudi Arabia.
Conflict of Interest
The authors declare that the research was conducted in the absence of any commercial or financial relationships that could be construed as a potential conflict of interest.
Publisher’s Note
All claims expressed in this article are solely those of the authors and do not necessarily represent those of their affiliated organizations, or those of the publisher, the editors and the reviewers. Any product that may be evaluated in this article, or claim that may be made by its manufacturer, is not guaranteed or endorsed by the publisher.
Acknowledgments
Authors would like to thank King Abdullah University of Science and Technology (KAUST), Saudi Arabia for the support.
Footnotes
1Please note that ϵo is same as ϵ, related to the severity of pointing error. However, ϵo shows the severity of pointing error of the inter-HAP links which is different than ϵ related to the ES-HAP link’s pointing error severity because different selection parameters are considered for both the links.
References
Ahmad, I., Nguyen, K. D., and Letzepis, N. (2017). “Performance Analysis of High Throughput Satellite Systems with Optical Feeder Links,” in IEEE Global Commun. Conf. (GLOBECOM), Singapore, 4–8 Dec 2017 (IEEE), 1–7. doi:10.1109/glocom.2017.8255105
Antonini, M., Betti, S., Carrozzo, V., Duca, E., and Ruggieri, M. (2006). “Feasibility Analysis of a HAP-LEO Optical Link for Data Relay Purposes,” in IEEE Aero. Conf., Big Sky, MT, USA, 4–11 March 2006 (IEEE), 1–7.
Ashrafzadeh, B., Zaimbashi, A., Soleimani-Nasab, E., and Uysal, M. (2020). Unified Performance Analysis of Multi-Hop FSO Systems over Double Generalized Gamma Turbulence Channels with Pointing Errors. IEEE Trans. Wireless Commun. 19, 7732–7746. doi:10.1109/twc.2020.3015780
Beckmann, P., and Spizzichino, A. (1987). The Scattering of Electromagnetic Waves from Rough Surfaces. Norwood, MA, USA: Artech House Radar Library; Artech Print on Demand.
Bhatnagar, M. R., and Arti, M. K. (2013). Performance Analysis of Hybrid Satellite-Terrestrial FSO Cooperative System. IEEE Photon. Technol. Lett. 25, 2197–2200. doi:10.1109/lpt.2013.2282836
Chen, J., Yang, L., Wang, W., Yang, H.-C., Liu, Y., Hasna, M. O., et al. (2019). A Novel Energy Harvesting Scheme for Mixed FSO-RF Relaying Systems. IEEE Trans. Veh. Technol. 68, 8259–8263. doi:10.1109/tvt.2019.2925736
Datsikas, C. K., Peppas, K. P., Sagias, N. C., and Tombras, G. S. (2010). Serial Free-Space Optical Relaying Communications over Gamma-Gamma Atmospheric Turbulence Channels. J. Opt. Commun. Netw. 2, 576–586. doi:10.1364/jocn.2.000576
David, F., Giggenbach, D., Henniger, H., Horwath, J., Landrock, R., and Perlot, N. (2004). “Design Considerations for Optical Inter-HAP Links,” in AIAA Int. Commun. Satellite Syst. Conf. Exhibit (ICSSC), 3144. doi:10.2514/6.2004-3144
d’Oliveira, F. A., Melo, F. C. L. D., and Devezas, T. C. (2016). High-Altitude Platforms—Present Situation and Technology Trends. J. Aero. Technol. Manage. 8, 249–262. doi:10.5028/jatm.v8i3.699
Farid, A. A., and Hranilovic, S. (2007). Outage Capacity Optimization for Free-Space Optical Links with Pointing Errors. J. Lightwave Technol. 25, 1702–1710. doi:10.1109/jlt.2007.899174
Fidler, F., Knapek, M., Horwath, J., and Leeb, W. R. (2010). Optical Communications for High-Altitude Platforms. IEEE J. Select. Top. Quan. Electron. 16, 1058–1070. doi:10.1109/jstqe.2010.2047382
Gao, N., Jin, S., Li, X., and Matthaiou, M. (2021). Aerial RIS-Assisted High Altitude Platform Communications. IEEE Wireless Commun. Lett. 10, 2096–2100. doi:10.1109/lwc.2021.3091164
Gradshteyn, I., and Ryzhik, I. (2000). Table of Integrals, Series and Products. 6th ed. New York, NY, USA: Academic.
ITU-Radiocommun (2000). Preferred Characteristics of Systems in the Fixed Service Using High Altitude Platforms Operating in the Bands 47.2-47.5 GHz and 47.9-48.2 GHz. Geneva: International Telecommunication Union, Electronic Publication. https://www.itu.int/dms_pubrec/itu-r/rec/f/R-REC-F.1500-0-200005-I!!PDF-E.pdf.
Jung, K.-J., Nam, S. S., Alouini, M.-S., and Ko, Y.-C. (2020). Unified Finite Series Approximation of FSO Performance Over Strong Turbulence Combined with Various Pointing Error Conditions. IEEE Trans. Commun. 68, 6413–6425. doi:10.1109/tcomm.2020.3008459
Kong, H., Lin, M., Zhu, W.-P., Amindavar, H., and Alouini, M.-S. (2020). Multiuser Scheduling for Asymmetric FSO/RF Links in Satellite-UAV-Terrestrial Networks. IEEE Wireless Commun. Lett. 9, 1235–1239. doi:10.1109/lwc.2020.2986750
Kurt, G. K., Khoshkholgh, M. G., Alfattani, S., Ibrahim, A., Darwish, T. S. J., Alam, M. S., et al. (2021). A Vision and Framework for the High Altitude Platform Station (HAPS) Networks of the Future. IEEE Commun. Surv. Tutorials 23, 729–779. doi:10.1109/comst.2021.3066905
Lee, J.-H., Park, K.-H., Ko, Y.-C., and Alouini, M.-S. (2020). Throughput Maximization of Mixed FSO/RF UAV-Aided Mobile Relaying with a Buffer. IEEE Trans. Wireless Commun. 20, 683–694. doi:10.1109/TWC.2020.3028068
Li, R., Chen, T., Fan, L., and Dang, A. (2019). Performance Analysis of a Multiuser Dual-Hop Amplify-And-Forward Relay System with FSO/RF Links. J. Opt. Commun. Netw. 11, 362–370. doi:10.1364/jocn.11.000362
Liu, X., Lin, M., Zhu, W.-P., Wang, J.-Y., and Upadhyay, P. K. (2020). Outage Performance for Mixed FSO-RF Transmission in Satellite-Aerial- Terrestrial Networks. IEEE Photon. Technol. Lett. 32, 1349–1352. doi:10.1109/lpt.2020.3025452
Michailidis, E. T., Nomikos, N., Bithas, P., Vouyioukas, D., and Kanatas, A. G. (2018). “Outage Probability of Triple-Hop Mixed RF/FSO/RF Stratospheric Communication Systems,” in Proc. IEEE Int. Conf. Adv. Satellite Space Commun. (SPACOMM), Athens, Greece, April 22–26, 2018, 1–6.
Milas, V., Koletta, M., and Constantinou, P. (2003). Interference and Compatibility Studies Between Satellite Service Systems and Systems Using High Altitude Platform Stations. ESA Spec. Publ. 541, 34.
Miura, R., and Oodo, M. (2001). Wireless Communications System Using Stratospheric Platforms: R and D Program on Telecom and Broadcasting System Using High Altitude Platform Stations. J. Commun. Res. Lab. 48, 33–48.
Mozaffari, M., Saad, W., Bennis, M., Nam, Y.-H., and Debbah, M. (2019). A Tutorial on UAVs for Wireless Networks: Applications, Challenges, and Open Problems. IEEE Commun. Surv. Tutorials 21, 2334–2360. doi:10.1109/comst.2019.2902862
Parthasarathy, S., Giggenbach, D., and Kirstädter, A. (2014). “Channel Modelling for Free-Space Optical Inter-HAP Links Using Adaptive ARQ Transmission,” in Unmanned/Unattended Sensors and Sensor Networks X (International Society for Optics and Photonics), Amsterdam, Netherlands, 17 October 2014, 9248, 92480Q. doi:10.1117/12.2067195
Saleh Altubaishi, E., and Alhamawi, K. (2019). Capacity Analysis of Hybrid AF Multi-Hop FSO/RF System Under Pointing Errors and Weather Effects. IEEE Photon. Technol. Lett. 31, 1304–1307. doi:10.1109/lpt.2019.2926679
Sharma, M., Chadha, D., and Chandra, V. (2016). High-Altitude Platform for Free-Space Optical Communication: Performance Evaluation and Reliability Analysis. J. Opt. Commun. Netw. 8, 600–609. doi:10.1364/jocn.8.000600
Sharma, S., Madhukumar, A. S., and R., S. (2019). Switching-Based Cooperative Decode-And-Forward Relaying for Hybrid FSO/RF Networks. J. Opt. Commun. Netw. 11, 267–281. doi:10.1364/jocn.11.000267
Shibata, Y., Kanazawa, N., Konishi, M., Hoshino, K., Ohta, Y., and Nagate, A. (2020). System Design of Gigabit HAPS Mobile Communications. IEEE Access 8, 157995–158007. doi:10.1109/access.2020.3019820
Singya, P. K., and Alouini, M.-S. (2021). Performance of UAV Assisted Multiuser Terrestrial-Satellite Communication System Over Mixed FSO/RF Channels. IEEE Trans. Aero. Electron. Syst. doi:10.1109/taes.2021.3111787
Singya, P. K., Kumar, N., Bhatia, V., and Alouini, M.-S. (2019). On Performance of Hexagonal, Cross, and Rectangular QAM for Multi-Relay Systems. IEEE Access 7, 60602–60616. doi:10.1109/access.2019.2915375
Singya, P. K., Kumar, N., Bhatia, V., and Alouini, M.-S. (2020). On the Performance Analysis of Higher Order QAM Schemes Over Mixed RF/FSO Systems. IEEE Trans. Veh. Technol. 69, 7366–7378. doi:10.1109/tvt.2020.2990747
Singya, P. K., Kumar, N., and Bhatia, V. (2018). Impact of Imperfect CSI on ASER of Hexagonal and Rectangular QAM for AF Relaying Network. IEEE Commun. Lett. 22, 428–431. doi:10.1109/lcomm.2017.2778153
Swaminathan, R., Sharma, S., Vishwakarma, N., and Madhukumar, A. (2021). HAPS-Based Relaying for Integrated Space-Air-Ground Networks with Hybrid FSO/RF Communication: A Performance Analysis. IEEE Trans. Aero. Electron. Syst. 57, 1581–1599. doi:10.1109/TAES.2021.3050663
Trichili, A., Cox, M. A., Ooi, B. S., and Alouini, M.-S. (2020). Roadmap to Free Space Optics. J. Opt. Soc. Am. B 37, A184–A201. doi:10.1364/josab.399168
Trichili, A., Ragheb, A., Briantcev, D., Esmail, M. A., Altamimi, M., Ashry, I., et al. (2021). Retrofitting FSO Systems in Existing RF Infrastructure: A Non-Zero-Sum Game Technology. IEEE Open J. Commun. Soc. 2, 2597–2615. doi:10.1109/ojcoms.2021.3130645
Vaiopoulos, N., Sandalidis, H. G., and Varoutas, D. (2013). Using a HAP Network to Transfer WiMAX OFDM Signals: Outage Probability Analysis. J. Opt. Commun. Netw. 5, 711–721. doi:10.1364/jocn.5.000711
Wang, P., Cao, T., Guo, L., Wang, R., and Yang, Y. (2015). Performance Analysis of Multihop Parallel Free-Space Optical Systems Over Exponentiated Weibull Fading Channels. IEEE Photon. J. 7, 1–17. doi:10.1109/jphot.2015.2396115
Wolframe (1998). The Wolfram Function Site. Available at: http://functions.wolfram.com/.
Xu, G., and Song, Z. (2020). Performance Analysis for Mixed κ-μ Fading and M-Distribution Dual-Hop Radio Frequency/Free Space Optical Communication Systems. IEEE Trans. Wireless Commun. 20, 1517–1528. doi:10.1109/TWC.2020.3034104
Xu, G., and Song, Z. (2021). Performance Analysis of a UAV-Assisted RF/FSO Relaying Systems for Internet of Vehicles. IEEE Internet Things J. doi:10.1109/JIOT.2021.3051211
Xu, G., and Zhang, Q. (2021). Mixed RF/FSO Deep Space Communication System Under Solar Scintillation Effect. IEEE Trans. Aero. Electron. Syst. 57, 3237–3251. doi:10.1109/taes.2021.3074130
Yang, L., Yuan, J., Liu, X., and Hasna, M. O. (2018). On the Performance of LAP-Based Multiple-Hop RF/FSO Systems. IEEE Trans. Aero. Electron. Syst. 55, 499–505. doi:10.1109/TAES.2018.2852399
Zedini, E., and Alouini, M.-S. (2015). Multihop Relaying over IM/DD FSO Systems with Pointing Errors. J. Lightwave Technol. 33, 5007–5015. doi:10.1109/jlt.2015.2492244
Zedini, E., Kammoun, A., and Alouini, M.-S. (2020). Performance of Multibeam Very High Throughput Satellite Systems Based on FSO Feeder Links with HPA Nonlinearity. IEEE Trans. Wireless Commun. 19, 5908–5923. doi:10.1109/twc.2020.2998139
Zedini, E., Soury, H., and Alouini, M.-S. (2016). On the Performance Analysis of Dual-Hop Mixed FSO/RF Systems. IEEE Trans. Wireless Commun. 15, 3679–3689. doi:10.1109/twc.2016.2524685
Zhan, P., Yu, K., and Swindlehurst, A. L. (2011). Wireless Relay Communications with Unmanned Aerial Vehicles: Performance and Optimization. IEEE Trans. Aerosp. Electron. Syst. 47, 2068–2085. doi:10.1109/taes.2011.5937283
Appendix
We have considered the Gamma-Gamma distribution for the atmospheric turbulence modeling of the ES-HAP FSO link and Meijer-G function is the prime element in the analytical expression. Hence, for asymptotic analysis, the meijer-G function must be approximated at high SNR which can be represented by the basic elementary form as (Wolframe, 1998, (07.34.06.0001.01))
where ϱ3 = [1, ϱ1] and ϱ4 = [ϱ2, 0]. As the power on the transmit SNR term decides the diversity order, the dominant term from (34) can be obtained as
From (35), it is observed that the diversity order of the RF link is U × m × Nt which directly depends on the number of terrestrial MUs (U), number of antennas (Nt), and RF link’s fading severity m. Finally, substituting (34) and (35) in (29), asymptotic outage probability for the considered system is obtained as (30).
Keywords: free-space optics, high altitude platform, gamma-gamma fading, pointing error, outage probability, average bit-error-rate
Citation: Singya PK and Alouini M-S (2022) Mixed FSO/RF Based Multiple HAPs Assisted Multiuser Multiantenna Terrestrial Communication. Front. Comms. Net 3:746201. doi: 10.3389/frcmn.2022.746201
Received: 23 July 2021; Accepted: 25 February 2022;
Published: 20 May 2022.
Edited by:
Steve Hranilovic, McMaster University, CanadaReviewed by:
Guanjun Xu, East China Normal University, ChinaMilica Petkovic, University of Novi Sad, Serbia
Copyright © 2022 Singya and Alouini. This is an open-access article distributed under the terms of the Creative Commons Attribution License (CC BY). The use, distribution or reproduction in other forums is permitted, provided the original author(s) and the copyright owner(s) are credited and that the original publication in this journal is cited, in accordance with accepted academic practice. No use, distribution or reproduction is permitted which does not comply with these terms.
*Correspondence: Praveen Kumar Singya, praveen.singya@kaust.edu.sa